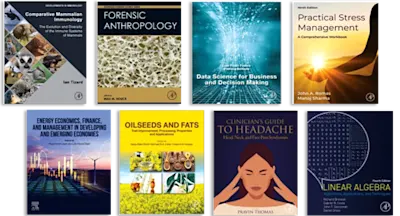
Thermoelectric Energy Conversion
Theories and Mechanisms, Materials, Devices, and Applications
- 1st Edition - January 19, 2021
- Imprint: Woodhead Publishing
- Editor: Ryoji Funahashi
- Language: English
- Paperback ISBN:9 7 8 - 0 - 1 2 - 8 1 8 5 3 5 - 3
- eBook ISBN:9 7 8 - 0 - 1 2 - 8 1 9 9 1 6 - 9
Thermoelectric Energy Conversion: Theories and Mechanisms, Materials, Devices, and Applications provides readers with foundational knowledge on key aspects of thermoelectric conver… Read more
Purchase options
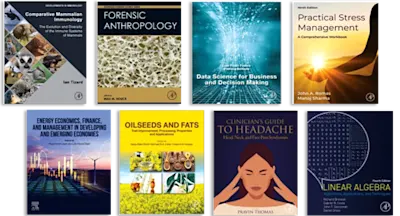
Institutional subscription on ScienceDirect
Request a sales quote- Reviews key applications of thermoelectric energy conversion, including cooling, power generation, energy harvesting, and applications for space and sensing
- Discusses a wide range of materials, including skutterudites, heusler materials, chalcogenides, oxides, low dimensional materials, and organic materials
- Provides the fundamentals of thermoelectric energy conversion, including the physics, phonon conduction, electronic correlation, magneto-seebeck theories, topological insulators and thermionics
Materials Scientists, Electrical and Thermal Engineers, Researchers in both academia and R&D
Section A: Theory and mechanism
1.1 Thermoelectric properties beyond the standard Boltzmann model in oxides: A focus on the ruthenates
Florent Pawula, Ramzy Daou, Sylvie He'bert, and Antoine Maignan
1.2 Electron correlation
Ichiro Terasaki
1.3 Thermal transport by phonons in thermoelectrics
Yuxuan Liao, Harsh Chandra, and Junichiro Shiomi
Section B: Materials
2.1 Bismuth telluride
Yu Pan and Jing-Feng Li
2.2 Thermoelectric properties of skutterudites
Ctirad Uher
2.3 Recent developments in half-Heusler thermoelectric materials
Jan-Willem G. Bos
2.4 Pseudogap engineering of Fe2VAl-based thermoelectric Heusler compounds
Yoichi Nishino
2.5 Zintl phases for thermoelectric applications
Susan M. Kauzlarich, Kasey P. Devlin, and Christopher J. Perez
2.6 High-performance sulfide thermoelectric materials
Anthony V. Powell
2.7 Synthetic minerals tetrahedrites and colusites for thermoelectric power generation
Koichiro Suekuni, Michihiro Ohta, Toshiro Takabatake, and Emmanuel Guilmeau
2.8 High-performance thermoelectrics based on metal selenides
Tanmoy Ghosh, Moinak Dutta, and Kanishka Biswas
2.9 Materials development and module fabrication in highly efficient lead tellurides
Michihiro Ohta, Priyanka Jood, Raju Chetty, and Mercouri G. Kanatzidis
2.10 Oxide thermoelectric materials: Compositional, structural, microstructural, and processing challenges to realize their potential
Slavko Bernik
2.11 Oxide thermoelectric materials
Dursun Ekren, Feridoon Azough, and Robert Freer
2.12 Thermoelectric materials-based on organic semiconductors
Qingshuo Wei, Masakazu Mukaida, Kazuhiro Kirihara, and Takao Ishida
2.13 Organic thermoelectric materials and devices
Hong Wang and Choongho Yu
2.14 Thermoelectric materials and devices based on carbon nanotubes
Yoshiyuki Nonoguchi
2.15 Higher manganese silicides
Yuzuru Miyazaki
2.16 Silicide materials: Thermoelectric, mechanical properties, and durability for Mg-Si and Mn-Si
Tsutomu Iida, Ryo Inoue, Daishi Shiojiri, Naomi Hirayama, Noriaki Hamada, and Yasuo Kogo
2.17 Highly efficient Mg2Si-based thermoelectric materials: A review on the micro- and nanostructure properties and the role of alloying
Georgios S. Polymeris, Euripides Hatzikraniotis, and Theodora Kyratsi
Section C: Devices and modules
3.1 Segmented modules
Shengqiang Bai, Qihao Zhang, and Lidong Chen
3.2 Power generation performance of Heusler Fe2VAl modules
Masashi Mikami
3.3 Microthermoelectric devices using Si nanowires
Takanobu Watanabe
3.4 Measurement techniques of thermoelectric devices and modules
Hsin Wang and Shengqiang Bai
3.5 Evaluation method and measurement example of thermoelectric devices and modules
Satoaki Ikeuchi
Section D: Applications
4.1 Thermoelectric air cooling
Kashif Irshad
4.2 Air-cooled thermoelectric generator
Ryoji Funahashi, Tomoyuki Urata, Yoko Matsumura, Hiroyo Murakami, and Hitomi Ikenishi
4.3 Prospects of TEG application from the thermoelectric cooling market
Hirokuni Hachiuma
4.4 Thermoelectric applications in passenger vehicles
Doug Crane
4.5 Thermoelectric generators for full-sized trucks and sports utility vehicles
James R Salvador
4.6 Thermoelectric generation using solar energy
Sajjad Mahmoudinezhad and Alireza Rezaniakolaei
4.7 Development and demonstration of outdoor-applicable thermoelectric generators for IoT applications
Kanae Nakagawa and Takashi Suzuki
- Edition: 1
- Published: January 19, 2021
- No. of pages (Paperback): 730
- Imprint: Woodhead Publishing
- Language: English
- Paperback ISBN: 9780128185353
- eBook ISBN: 9780128199169
RF