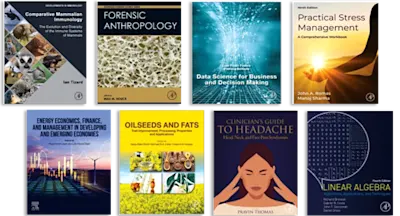
The Three Functional States of Proteins
Structured, Intrinsically Disordered, and Phase Separated
- 1st Edition - November 17, 2024
- Imprint: Academic Press
- Editors: Timir Tripathi, Vladimir N Uversky
- Language: English
- Paperback ISBN:9 7 8 - 0 - 4 4 3 - 2 1 8 0 9 - 5
- eBook ISBN:9 7 8 - 0 - 4 4 3 - 2 1 8 1 0 - 1
The Three Functional States of Proteins explores how structured proteins, intrinsically disordered proteins, and phase separated proteins contribute to the complexity of ce… Read more
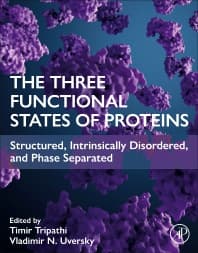
Purchase options
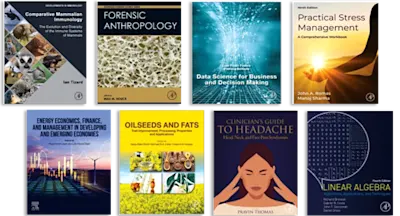
Institutional subscription on ScienceDirect
Request a sales quoteThe Three Functional States of Proteins explores how structured proteins, intrinsically disordered proteins, and phase separated proteins contribute to the complexity of cellular life, and offers insights into their roles in both health and disease. It discusses the latest research findings and highlight groundbreaking discoveries and innovative methodologies used to study these protein states.
Traditionally, the different states of proteins have been defined based on their structures and functions. However, it is becoming increasingly clear that these criteria alone may not be sufficient to capture the complex and multifaceted properties of these molecules. Definitions based on thermodynamics and kinetics are now recognized as potentially more appropriate for comprehensively understanding protein states. Emerging evidence indicates that under physiological conditions, a majority of proteins possess the capability to exist in and transition between the native, droplet, and amyloid states. These distinct states play crucial roles in various cellular functions, influenced significantly by their physicochemical and structural properties. The book also considers the interactions among these states and discusses how their internal organization as individual molecules, as well as their collective organization as molecular assemblies are stabilized. Furthermore, it examines the processes by which these states are formed and the cellular functions associated with each specific state.
- The book serves as an introduction to a unique volume that provides comprehensive coverage of these three functional states of proteins
- The chapters are written by leading global scientists who are actively engaged in research on these specific protein states
- It presents a broad picture of the current, emerging, and evolving research on these protein states
- Given that this book comprehensively addresses both foundational concepts and recent advancements in the field, it will appeal a broad spectrum of readers from various academic disciplines
Biophysicists, Structural biologists, Bioinformatics and Computational biologists, Biochemists, Pharmaceutical scientists, Medicinal chemists, and Researchers in both academia and industry, PhD students, senior undergraduate students and postdoctoral fellows
- Title of Book
- Cover image
- Title page
- Table of Contents
- Copyright
- List of contributors
- Preface
- Chapter 1. The three functional states of proteins: beyond the classical “lock and key” paradigm
- Abstract
- 1.1 Structure-function continuum at the ground level: linking functional order and disorder
- 1.2 Functionality in 1D: functional amyloids
- 1.3 Protein quinary structure: membrane-less organelles and biomolecular condensates
- References
- Chapter 2. Ordered proteins and structure–function relationship: a classical view
- Abstract
- 2.1 Protein structure: classical view
- 2.2 Tools and techniques which fashioned the classical enzymology
- 2.3 Chemical modification, chemical cross-linking, bioconjugation
- 2.4 RNase A and RNase S as evergreen models for exploring structure–function relationships
- 2.5 Conclusions
- References
- Chapter 3. Binding of a substrate (“lock and key”) and conformational adaption (“induced fit”) are different stages of enzyme action
- Abstract
- 3.1 Introduction
- 3.2 The concept of “native structure” and its evolution
- 3.3 Protein dynamics: moving away from the “rigid lock” concept
- 3.4 Induced fit: a legacy of Emil-Fischer’s ideas
- 3.5 Enzymes/proteins, which conform to the induced fit mechanism
- 3.6 “Conformational selection” model: not quite like looking for “a needle in a haystack”
- 3.7 Distinguishing between the “induced folding” and “conformational selection” mechanisms
- 3.8 What model is followed by intrinsically disordered proteins/intrinsically disordered regions?
- 3.9 Conclusions
- References
- Chapter 4. Intrinsically disordered proteins: functionality of chaos
- Abstract
- 4.1 Introduction
- 4.2 Types of IDPs
- 4.3 Mechanisms of IDP function
- 4.4 Biological importance of IDPs
- 4.5 Methods to study IDPs
- 4.6 Conclusion
- Acknowledgment
- References
- Chapter 5. Protein conformation-based phenotypic switching and implications in the origin and evolution of multicellularity
- Abstract
- 5.1 Introduction
- 5.2 Correlation between protein disorder and biological complexity
- 5.3 Noise and phenotypic switching
- 5.4 IDPs, prion-like IDPs, and protein conformation-based phenotypic switching
- 5.5 Stochastic mathematical models to delve into the dynamics of multicellularity
- 5.6 Conclusions and future directions
- Conflict of Interest
- References
- Chapter 6. Hybrid proteins: fusion chimeras and natural wonders
- Abstract
- 6.1 Introduction
- 6.2 Chimeric proteins generated by gene translocation
- 6.3 Grafting of functional peptides
- 6.4 Structure–function relationship of hybrid proteins
- 6.5 Exploring the functional significance of hybrid proteins
- 6.6 Hybrid proteins, containing ordered and disordered regions
- 6.7 Methods used to study hybrid proteins
- 6.8 Examples and case studies of hybrid proteins
- 6.9 Conclusion and future directions
- References
- Chapter 7. Functional protein oligomers
- Abstract
- 7.1 Introduction
- 7.2 Types of protein oligomers
- 7.3 Symmetry and asymmetry in protein oligomers
- 7.4 Types of functional oligomers
- 7.5 Role of intrinsic disorder in oligomer formation
- 7.6 Regulation of protein oligomers
- 7.7 Protein oligomers in health and disease
- 7.8 Conclusion
- Acknowledgments
- References
- Chapter 8. Fuzzy complexes
- Abstract
- 8.1 Introduction
- 8.2 Classification
- 8.3 Biological functions
- 8.4 Pathology and druggability
- 8.5 Experimental and computational characterization
- 8.6 Conclusion
- Acknowledgments
- References
- Chapter 9. SMARTQ: single-molecule amyloid fibRil tracking and quantification. A method for accurately imaging, tracking, and quantifying the growth of individual amyloid fibrils using TIRF
- Abstract
- 9.1 Introduction
- 9.2 The SMARTQ procedure
- 9.3 Conclusion
- Acknowledgments
- Abbreviations
- References
- Chapter 10. Structural polymorphism in amyloids—states within proteins’ solid-state
- Abstract
- 10.1 Biochemistry and biophysics of protein aggregation and amyloid formation
- 10.2 Polymorphism and phenotypes
- 10.3 Origins of polymorphic amyloid fibrils
- 10.4 Aβ
- 10.5 Tau
- 10.6 α-Synuclein
- 10.7 TDP-43
- 10.8 Polymorphism via heterotypic protein–protein interaction—a new frontier in amyloid polymorphism
- 10.9 Conclusions
- References
- Chapter 11. Liquid–liquid phase separation, biomolecular condensates, and membraneless organelles: a novel blueprint of intracellular organization
- Abstract
- 11.1 Introduction
- 11.2 Liquid–liquid phase separation in biomolecular condensates
- 11.3 Interactions in LLPS, biomolecular condensates, and MLOs
- 11.4 Regulation of formation of biomolecular condensates
- 11.5 Roles of biomolecular condensates and MLOs in diseases
- 11.6 LLPS, biomolecular condensates, and MLOs: opportunities for drug discovery and therapeutic interventions
- 11.7 Imaging and visualization techniques for studying LLPS, biomolecular condensates, and MLOs
- 11.8 Challenges in studying LLPS, biomolecular condensates, and MLOs
- 11.9 Future prospects and implications
- 11.10 Conclusions
- References
- Chapter 12. Physical principles and molecular interactions underlying protein phase separation
- Abstract
- 12.1 Introduction to protein phase separation
- 12.2 Thermodynamics and driving forces of protein phase separation
- 12.3 Molecular interactions in protein phase separation
- 12.4 Regulation of phase separation
- 12.5 Functional implications of protein phase separation
- 12.6 Targeting protein phase separation for drug discovery
- 12.7 Conclusion and future directions
- References
- Chapter 13. Various levels of phase transitions in the protein universe and around
- Abstract
- 13.1 Introduction
- 13.2 Phases and phase transitions
- 13.3 Intramolecular phase transitions in disordered proteins induced by interactions with binding partners
- 13.4 Proteins that catalyze and inhibit phase transitions: ice nucleation and antinucleation
- 13.5 Protein amorphous aggregation, fibrillation, and crystallization as intermolecular phase transitions
- 13.6 Reincarnation of liquid–liquid and liquid–gel phase transitions: drivers of the biogenesis of membrane-less organelles and biomolecular condensates
- 13.7 Conclusions
- Acknowledgments
- Author Contributions
- Funding
- Conflict of Interest
- References
- Chapter 14. Targeting phase separated protein states for drug discovery
- Abstract
- 14.1 Introduction
- 14.2 Biophysics underlying protein phase separation
- 14.3 Diseases associated with protein phase separation
- 14.4 Strategies for targeting phase separated protein states for drug discovery
- 14.5 Targeting phase separated protein states for drug discovery
- 14.6 Future directions and emerging technologies to assist in targeting phase separated states for drug discovery
- 14.7 Conclusions and future prospects
- References
- Chapter 15. Protein hydrogels: structure, characteristics, and applications
- Abstract
- 15.1 Introduction
- 15.2 Crosslinking strategies in hydrogel production
- 15.3 Categories of protein hydrogels
- 15.4 Applications of protein hydrogels
- 15.5 Challenges of protein hydrogel applications and future directions
- 15.6 Conclusions
- References
- Chapter 16. Interactions among the three protein states
- Abstract
- 16.1 Theoretical background
- 16.2 High-resolution structure determination
- 16.3 Nuclear magnetic resonance
- 16.4 Hydrodynamic analysis
- Acknowledgments
- References
- Chapter 17. Frustration and fuzziness in the three functional states of proteins
- Abstract
- 17.1 Introduction
- 17.2 Energy landscape theory of protein folding
- 17.3 Evolutionary perspectives on frustration and fuzziness
- 17.4 Frustration and fuzziness in phase-separated protein assemblies
- 17.5 Experimental approaches to probing frustration and fuzziness in distinct functional states
- 17.6 Computational approaches to investigating fuzziness in different states
- 17.7 Conclusion
- Acknowledgments
- References
- Chapter 18. Thermoresponsive intrinsically disordered protein polymers
- Abstract
- 18.1 Introduction
- 18.2 Elastin-like polypeptides
- 18.3 Resilin-like polypeptides
- 18.4 Silk-elastin-like proteins
- 18.5 Glutamine-rich polypeptides (Q-rich polypeptides)
- 18.6 Collagen-like polypeptides
- 18.7 Analysis and characterization of TIDPPs
- 18.8 Thermally responsive disordered polymer segments: a solubilization strategy based on entropic flexibility
- 18.9 Future directions for TIDDPs research
- 18.10 Conclusions
- References
- Chapter 19. The evolution and exploration of intrinsically disordered and phase-separated protein states
- Abstract
- 19.1 What are intrinsically disordered regions?
- 19.2 IDRs across the tree of life
- 19.3 IDRs and the origin of proteins
- 19.4 Evolutionary selection of IDRs
- 19.5 Conserved sequence features of IDRs confer function
- 19.6 Phase separation as an emerging function of IDRs
- 19.7 Our knowledge of IDR evolution is limited to “alignable disorder”
- 19.8 Unalignable disorder—emerging methods to study IDR evolution
- 19.9 Conclusion and outlook
- Acknowledgments
- References
- Chapter 20. Computational modeling of intrinsically disordered and phase-separated protein states
- Abstract
- 20.1 Introduction
- References
- Chapter 21. Molecular dynamics simulations of intrinsically disordered proteins, fuzzy complexes, and phase-separated protein states
- Abstract
- 21.1 Introduction
- 21.2 Insights into IDPs/IDRs from MD simulations
- 21.3 Insights into fuzzy complexes from MD simulations
- 21.4 Insights into phase-separation of proteins from MD simulation
- 21.5 Conclusions
- Acknowledgments
- References
- Chapter 22. Biological complexity of the phase-separated protein states
- Abstract
- 22.1 Introduction
- 22.2 Composition of biomolecular condensates: complexity of structural organization
- 22.3 Types of biomolecular condensates and related transitions
- 22.4 Characteristics of the biomolecular condensates: compartmentalization, localization, and regulation
- 22.5 Properties of phase-separated condensates
- 22.6 Peculiarities of the amino acid sequences of proteins engaged in phase separation
- 22.7 Structural and dynamic features of biomolecular condensates
- 22.8 Biomolecular condensates in diseases
- 22.9 Conclusions
- Authors contributions
- References
- Chapter 23. Protein structure–function continuum
- Abstract
- 23.1 Structure–function continuum at the individual molecule level
- 23.2 Structure–function continuum and functional amyloids
- 23.3 Structure–function continuum and membrane-less organelles
- 23.4 Structure–function continuum and the interplay between the three functional states of proteins: intrinsic disorder as one ring to rule them all
- References
- Index
- Edition: 1
- Published: November 17, 2024
- Imprint: Academic Press
- No. of pages: 474
- Language: English
- Paperback ISBN: 9780443218095
- eBook ISBN: 9780443218101
TT
Timir Tripathi
Professor Timir Tripathi is a Professor of Molecular Biology, School of Life Sciences, North-Eastern Hill University, Shillong, India. Earlier, he served as the Regional Director of Indira Gandhi National Open University. His previous role was Senior Assistant Professor and Principal Investigator at the Department of Biochemistry, NEHU, Shillong. He holds a Ph.D. from Jawaharlal Nehru University, New Delhi. His primary research focus is studying the conformational dynamics, interaction, and stabilization of the complexes formed by intrinsically disordered neuropathological protein aggregates, their properties of liquid-liquid phase separation, interaction, and roles in nucleocytoplasmic transport in neurodegenerative diseases. Professor Tripathi is an Associate Fellow of the Indian National Science Academy, New Delhi, and an elected member of the National Academy of Sciences, India, the Royal Society of Chemistry, and the Royal Society of Biology, UK
VU
Vladimir N Uversky
Prof. Vladimir N. Uversky, PhD, DSc, FRSB, FRSC, F.A.I.M.B.E., Professor at the Department of Molecular Medicine, Morsani College of Medicine, University of South Florida (USF), is a pioneer in the field of protein intrinsic disorder. He has made a number of groundbreaking contributions in the field of protein folding, misfolding, and intrinsic disorder. He obtained his academic degrees from Moscow Institute of Physics and Technology (Ph.D., in 1991) and from the Institute of Experimental and Theoretical Biophysics, Russian Academy of Sciences (D.Sc., in 1998). He spent his early career working mostly on protein folding at the Institute of Protein Research and the Institute for Biological Instrumentation (Russia). In 1998, moved to the University of California Santa Cruz. In 2004, joined the Indiana University−Purdue University Indianapolis as a Senior Research Professor. Since 2010, Professor Uversky is with USF, where he works on various aspects of protein intrinsic disorder phenomenon and on analysis of protein folding and misfolding processes. Prof. Uversky has authored over 1250 scientific publications and edited several books and book series on protein structure, function, folding, misfolding, and intrinsic disorder. He is also serving as an editor in a number of scientific journals. He was a co-founder of the Intrinsically Disordered Proteins Subgroup at the Biophysical Society and the Intrinsically Disordered Proteins Gordon Research Conference. Prof. Uversky collaborated with more than 12,500 colleagues from more than 2,750 research organizations in 89 countries/territories.