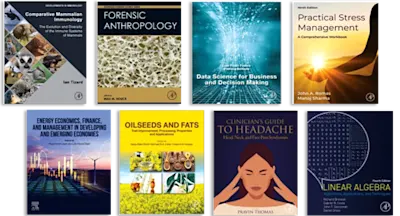
The Theory and Practice of Scintillation Counting
International Series of Monographs in Electronics and Instrumentation
- 1st Edition - January 1, 1964
- Imprint: Pergamon
- Author: J. B. Birks
- Editors: D.W. Fry, L. Costrell, K. Kandiah
- Language: English
- Hardback ISBN:9 7 8 - 0 - 0 8 - 0 1 0 4 7 2 - 0
- Paperback ISBN:9 7 8 - 1 - 4 8 3 1 - 2 3 6 4 - 6
- eBook ISBN:9 7 8 - 1 - 4 8 3 1 - 5 6 0 6 - 4
The Theory and Practice of Scintillation Counting is a comprehensive account of the theory and practice of scintillation counting. This text covers the study of the scintillation… Read more
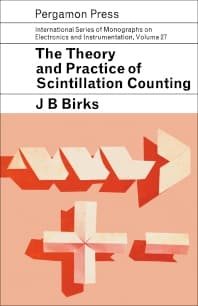
Purchase options
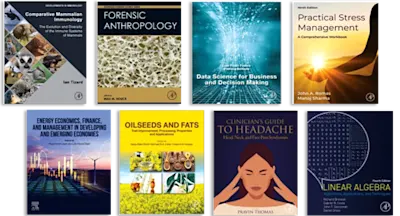
Institutional subscription on ScienceDirect
Request a sales quoteThe Theory and Practice of Scintillation Counting is a comprehensive account of the theory and practice of scintillation counting. This text covers the study of the scintillation process, which is concerned with the interactions of radiation and matter; the design of the scintillation counter; and the wide range of applications of scintillation counters in pure and applied science. The book is easy to read despite the complex nature of the subject it attempts to discuss. It is organized such that the first five chapters illustrate the fundamental concepts of scintillation counting. Chapters 6 to 10 detail the properties and applications of organic scintillators, while the next four chapters discuss inorganic scintillators. The last two chapters provide a review of some outstanding problems and a postscript. Nuclear physicists, radiation technologists, and postgraduate students of nuclear physics will find the book a good reference material.
Preface
Acknowledgments
Chapter 1. Introduction
1.1. The Detection of Atomic and Nuclear Radiations
1.1.1. Dosimeters
1.1.2. Track Visualization Instruments
1.1.3. Counters
1.1.4. Applications of Counters
1.2. Early History of the Scintillation Counter
1.2.1. Visual Scintillation Counters
1.2.2. Geiger Scintillation Counters
1.2.3. Photomultiplier Scintillation Counters
1.3. Principles of the Scintillation Counter
1.4. General Bibliography
1.5. References
Chapter 2. Absorption of the Incident Radiation
2.1. Nature of the Radiations
2.2. Heavy Charged Particles
2.3. Electrons
2.4. Electromagnetic Radiations
2.4.1. The Compton Effect
2.4.2. The Photo-electric Effect
2.4.3. Pair Production
2.4.4. Multiple Processes
2.5. Neutrons
2.5.1. Scattering
2.5.2. Absorption
2.6. References
Chapter 3. The Scintillation Process in Organic Materials—I
3.1. The Electronic Structure of Organic Molecules
3.2. Excited States of π-Electron Systems
3.2.1. Classification on Free-electron Model
3.2.2. Absorption Spectra
3.3. Luminescence
3.3.1. Fluorescence
3.3.2. Phosphorescence and Delayed Fluorescence
3.3.3. Dimers
3.4. Classification of Organic Scintillators
3.5. Outline of Scintillation Phenomena
3.6. The Scintillation Mechanism
3.7. The Primary Processes
3.7.1. Excitation and Ionization
3.7.2. Primary Excitation Energy
3.7.3. Internal Conversion
3.8. Fluorescence of Unitary Systems
3.8.1. De-excitation Processes
3.8.2. Thin Crystals
3.8.3. Thick Crystals
3.9. Energy Transfer and Fluorescence in Binary Systems
3.10. Energy Transfer and Fluorescence in Ternary Systems
3.11. The Absolute Scintillation Efficiency
3.12. References
Chapter 4. The Scintillation Process in Inorganic Crystals—I
4.1. Introduction
4.2. The Energy Band Model
4.2.1. Perfect Crystals
4.2.2. Imperfect Crystals
4.3. Conditions for Luminescence of a Center
4.4. Classification of Inorganic Phosphors and Scintillators
4.4.1. Phosphors
4.4.2. Scintillators
4.5. Outline of Scintillation Phenomena
4.6. Optical Properties of Alkali Halide Crystals
4.6.1. General
4.6.2. Absorption Spectra
4.6.3. Luminescence Spectra
4.7. Optical Properties of Thallium Activator Centers
4.7.1. Absorption Spectra
4.7.2. Luminescence Spectra
4.7.3. Theoretical Model
4.7.4. Photoluminescence Decay Times
4.8. The Scintillation Mechanism
4.8.1. Sequence of Processes
4.8.2. The Absolute Scintillation Efficiency
4.9. References
Chapter 5. The Detection of Scintillations
5.1. Light Collection
5.1.1. Self-absorption in the Scintillator
5.1.2. Light Trapping
5.1.3. Reflectors
5.1.4. NaI(Tl) Crystal Assemblies
5.1.5. Light Guides and Couplers
5.2. Spectral Response
5.2.1. Spectral Matching
5.2.2. Factors Determining Cathode Spectral Response
5.2.3. Specification of Cathode Response and Sensitivity
5.2.4. Types of Photocathode
5.3. Photomultipliers
5.3.1. Dynode Structures
5.3.2. Cathode-First Dynode Structures
5.3.3. Uniformity of Photocathode Response
5.3.4. Gain
5.3.5. High Tension Supply
5.3.6. The Anode
5.3.7. Feedback and Satellite Pulses
5.3.8. Other Background Effects
5.3.9. Fatigue
5.3.10. Magnetic Field Effects
5.3.11. Dark Noise
5.3.12. Reduction of Effect of Dark Noise
5.3.13. Temperature Dependence of Sensitivity and Response
5.3.14. Commercial Photomultipliers
5.4. Pulse Amplitude Resolution
5.4.1. Theoretical Studies
5.4.2. Factors Contributing to Line Width
5.4.3. Scintillator Resolution
5.4.4. Experimental Studies
5.4.5. Anthracene Excited by Electrons
5.4.6. Sodium Iodide (Thallium) Excited by γ-Rays
5.5. Pulse Shape and Time Resolution
5.5.1. The Light Pulse and Photo-electron Emission
5.5.2. The Single Electron Response
5.5.3. The Anode Pulse Shape
5.5.4. Statistics and Time Resolution
5.5.5. Effect of Anode Pulse Shape on Pulse Amplitude Resolution
5.6. References
Chapter 6. The Scintillation Process in Organic Materials—II
6.1. The Scintillation Response to Different Ionizing Radiations
6.1.1. Review of Experimental Data
6.1.2. Ionization Quenching
6.1.3. Bimolecular Quenching
6.1.4. Static and Dynamic Quenching
6.1.5. Response to Heavy Ions
6.1.6. Kinetics of Quenching
6.2. Surface Quenching Effects
6.3. Radiation Damage
6.3.1. Crystals
6.3.2. Plastic Solutions
6.3.3. Liquid Solutions
6.4. The Scintillation and Fluorescence Decay of Pure Crystals
6.4.1. Theory
6.4.2. Anthracene
6.4.3. Other Crystals
6.5. The Slow Scintillation Component
6.5.1. Review of Experimental Data
6.5.2. Theories of Origin
6.6. The Scintillation Decay of Solutions
6.6.1. Theory of Binary Solutions
6.6.2. Review of Experimental Data
6.6.3. Ternary Solutions
6.7. References
Chapter 7. Organic Crystal Scintillators
7.1. Introduction
7.2. Anthracene
7.2.1. Crystal Structure
7.2.2. Absorption Spectra
7.2.3. Anthracene as a Scintillator Standard
7.2.4. Purification and Crystal Growth
7.2.5. Scintillation Efficiency
7.2.6. Other Data
7.3. Other Organic Crystals
7.3.1. Relative Efficiencies
7.3.2. Scintillation Decay Times
7.3.3. trans-Stilbene
7.3.4. Diphenylacetylene
7.3.5. p-Terphenyl
7.3.6. p-Quaterphenyl
7.3.7. 1,4-Diphenylbutadiene
7.4. Mixed Organic Crystals and Exciton Migration
7.4.1. Review of Experimental Studies
7.4.2. Energy Transfer and Exciton Migration
7.4.3. Response Anisotropy
7.4.4. Exciton Lifetime
7.4.5. Low Temperature Behavior
7.4.6. Radiative Transfer
7.4.7. Mixed Crystal Scintillators
7.5. References
Chapter 8. Organic Liquid Scintillators
8.1. Introduction
8.2. Pure Solvents
8.3. Mixed Solvents and Loading with Other Compounds
8.3.1. Mixed Solvents
8.3.2. Naphthalene as an Additive
8.3.3. Boron Loading
8.3.4. Dioxane Mixtures
8.4. Primary Solutes
8.4.1. Initial Studies
8.4.2. The Oxazoles, Oxadiazoles and Related Compounds
8.4.3. The Substituted p-Oligophenylenes
8.5. Secondary Solutes
8.6. Spectral Effects
8.7. Oxygen Quenching
8.7.1. Experimental Studies
8.7.2. Elimination of Oxygen
8.8. Temperature Effects
8.9. Scintillation Decay Times
8.10. Energy Migration and Transfer
8.10.1. Initial Studies
8.10.2. Ultraviolet Excitation Studies
8.10.3. PPO-Xylene Solutions
8.10.4. Impurity Quenching Studies
8.10.5. Dipole-Dipole Interaction
8.10.6. Effect of Molecular Diffusion
8.10.7. Description of Solvent-Solute Transfer Process
8.10.8. The Domain Hypothesis
8.10.9. Solute-Solute Energy Transfer
8.11. Concentration Quenching and Dimer Formation
8.12. References
Chapter 9. Organic Plastic Scintillators
9.1. Introduction
9.2. Preparation of Plastic Scintillators
9.3. Solvents
9.3.1. Relative Efficiencies
9.3.2. Mixed Solvents
9.3.3. Influence of Molecular Weight
9.4. Primary and Secondary Solutes
9.5. Optical Transmission
9.6. Temperature Effects
9.7. Loading with Heavy Elements
9.8. Scintillation Decay Times
9.9. Organic Glasses
9.10. Energy Transfer
9.10.1. Radiative and Non-radiative Transfer
9.10.2. Non-radiative Transfer and Migration
9.10.3. Impurity Quenching Studies
9.10.4. Polymer Chain Attachment
9.10.5. Stereo-isomerism
9.11. Molecular Structure and Scintillation Properties
9.11.1. Solvents
9.11.2. Solutes
9.12. References
Chapter 10. Applications of Organic Scintillators
10.1. Properties and Uses of Organic Scintillators
10.2. β-Particle Detection and Spectrometry
10.2.1. β-Particle Detection
10.2.2. Internal-sample Liquid Scintillation Counting
10.2.3. Incorporation of the Specimen
10.2.4. Low-level Counting Systems
10.2.5. β-Particle Spectrometry
10.2.6. Scintillators in Magnetic Spectrometers
10.3. γ-Ray Detection and Spectrometry
10.3.1. γ-Ray Spectrometry
10.3.2. γ-Ray Detection
10.4. Fast Neutron Detection and Spectrometry
10.4.1. Single Scintillator Spectrometry
10.4.2. Neutron-γ-Ray Discrimination
10.4.3. Heterogeneous Scintillators for η/γ Discrimination
10.4.4. The "Twin" Fast Neutron Detector
10.4.5. Pulse Shape Discrimination
10.5. Coincidence Methods
10.5.1. Introduction
10.5.2. Coincidence Circuits and Timing Devices
10.5.3. Absolute Source Calibration
10.5.4. γ-Ray Angular Correlation and Polarization Studies
10.5.5. Positron Annihilation Studies
10.5.6. Delayed Coincidences and Lifetimes of Excited States of Nuclei
10.5.7. Coincidence β-Particle and γ-Ray Spectrometry
10.5.8. Coincidence Spectrometry of Fast Neutrons
10.5.9. Time-of-flight Spectrometry of Fast Neutrons
10.6. High Energy and Elementary Particle Studies
10.6.1. Large Scintillators
10.6.2. Counter Telescopes
10.6.3. Scintillation Chambers
10.6.4. Neutron and Neutrino Studies
10.7. References
Chapter 11. The Scintillation Process in Inorganic Crystals—II
11.1. The Scintillation Response to γ-Rays and Electrons
11.1.1. Sodium Iodide (Thallium)
11.1.2. Cesium Iodide (Thallium)
11.1.3. Discussion
11.2. The Scintillation Response to Heavy Ionizing Particles
11.2.1. Sodium Iodide (Thallium)
11.2.2. Effect of Tl Concentration
11.2.3. Cesium Iodide (Thallium)
11.2.4. Other Alkali Halides
11.3. Theory of the Scintillation Response
11.3.1. Review of the Data
11.3.2. The Murray-Meyer Model
11.3.3. Comparison with Organic Scintillators
11.3.4. The Effect of δ-Rays
11.4. The Scintillation Rise and Decay
11.4.1. Sodium Iodide (Thallium)
11.4.2. Temperature Dependence in NaI(Tl) and "Pure" NaI
11.4.3. Cesium Iodide (Thallium)
11.4.4. Other Alkali Halides
11.5. Effect of Temperature on Scintillation Efficiency
11.5.1. Theoretical Model
11.5.2. "Pure" Alkali Halides
11.5.3. Sodium Iodide (Thallium)
11.5.4. Cesium Iodide (Thallium)
11.5.5. An Approach to a General Theory
11.6. References
Chapter 12. Alkali Halide Crystals Scintillators and Their Applications
12.1. Sodium Iodide (Thallium)
12.1.1. Review of Previous Data
12.1.2. Crystal Preparation
12.1.3. Scintillation Efficiency
12.1.4. The Photon Interaction Ratio
12.1.5. Scattered Radiation
12.1.6. The Photon Escape Peaks
12.1.7. The Iodine Escape Peak
12.1.8. X-Ray Spectrometry
12.1.9. The Photo-fraction and y-Ray Detection Efficiency
12.1.10. γ-Ray Spectrometry
12.1.11. The Spectrometer Response Function
12.1.12. Pulse Amplitude Analyzers
12.1.13. Total Absorption and Summing Spectrometers
12.1.14. Anti-coincidence Shielding
12.1.15. Coincidence Spectrometers
12.1.16. Neutron Absorption
12.1.17. Human γ-Ray Spectrometry
12.1.18. Detection of High-energy γ-Rays and Electrons
12.1.19. Other Applications
12.2. Cesium Iodide (Thallium)
12.2.1. Review of Previous Data
12.2.2. Crystal Preparation
12.2.3. Scintillation Efficiency and Emission Spectrum
12.2.4. γ-Ray Spectrometry
12.2.5. Heavy Particle and Fast Neutron Spectrometry
12.2.6. Particle Discrimination
12.2.7. Dual Scintillator Systems
12.3. Lithium Iodide Phosphors
12.3.1. Slow Neutron Detection
12.3.2. Fast Neutron Spectrometry
12.4. Potassium Iodide (Thallium)
12.5. "Pure" Alkali Halides
12.5.1. Sodium Iodide
12.5.2. Cesium Iodide
12.5.3. Low Temperature Studies
12.6. Other Alkali Halides
12.6.1. Rubidium Iodide (Thallium)
12.6.2. Cesium Fluoride
12.6.3. Cesium Bromide (Thallium)
12.6.4. Other Materials
12.7. References
Chapter 13. Other Inorganic Solid Scintillators and Their Applications
13.1. Zinc Sulphide
13.1.1. Detection and Scintillation Efficiencies
13.1.2. Scintillation Decay
13.1.3. Heavy Particle Detection
13.1.4. Scintillation Response
13.1.5. Radiation Damage
13.1.6. Fast Neutron Detection
13.1.7. Slow Neutron Detection
13.2. Cadmium Sulphide
13.3. The Tungstate Phosphors
13.4. Inorganic Glass Scintillators
13.4.1. Composition and Scintillation Efficiency
13.4.2. Slow Neutron Detection
13.5. Other Inorganic Scintillators
13.5.1. Boron Compounds
13.5.2. Diamond
13.5.3. Scintillators and Phosphors Containing Various Elements
13.6. References
Chapter 14. Gas Scintillators and Their Applications
14.1. Nitrogen and Air
14.1.1. Introduction
14.1.2. Emission Spectrum
14.1.3. Scintillation Efficiency and Collisional Quenching
14.1.4. Scintillation Decay Times
14.1.5. Background Luminescence from Air, Glass and Quartz
14.2. The Inert Gases
14.2.1. Introduction
14.2.2. The Primary Processes
14.2.3. Emission Spectra
14.2.4. Scintillation Decay Times
14.2.5. The Effect of Nitrogen
14.2.6. Fluorescent Converters and Practical Scintillation Efficiencies
14.2.7. Mixtures of Inert Gases
14.2.8. Scintillation Response to Different Particles
14.2.9. Applications of Gas Scintillators
14.2.10. Light Amplification by an Electric Field
14.2.11. High-pressure Gas Scintillators
14.2.12. Neutron Detection and Spectrometry
14.3. Liquid and Solid Inert Elements
14.3.1. Scintillation Properties
14.3.2. Liquid Helium
14.3.3. Neutron Polarimetry
14.4. References
Chapter 15. Conclusion
References
Chapter 16. Postscript
3.7.1. The Primary Processes in Organic Materials
3.7.3. Internal Conversion in Organic Materials
5.1.5. Light Guides and Couplers
5.3.9. Photomultiplier Fatigue
5.3.12. Reduction of Effect of Dark Noise
5.3.14. Commercial Photomultipliers
5.4. Pulse Amplitude Resolution
5.5.4. Statistics and Time Resolution
5.5.5. Effect of Anode Pulse Shape on Pulse Amplitude Resolution
6.1. Scintillation Response of Anthracene to Different Ionizing Radiations
6.3.3. Radiation Damage of Liquid Solutions
6.4. The Scintillation Decay of Organic Crystals
6.5. The Slow Scintillation Component in Organic Systems
7.3. Scintillation and Fluorescence Efficiencies of Organic Crystals
7.4. Mixed Organic Crystals
7.4.3. Scintillation Response Anisotropy
8.2. Liquid Scintillator Solvents
8.4. Liquid Scintillator Solutes
8.9. Liquid Scintillator Decay Times
8.10. Energy Migration and Transfer
8.11. Dimer Formation
9.2. Preparation of Plastic Scintillators
9.8. Plastic Scintillator Decay Times
9.10. Energy Transfer in Plastic Scintillators
10.2. β-Particle Detection and Spectrometry
10.5. Coincidence Methods
11.1. Scintillation Response of NaI(Tl) and Cs(Tl) to X-Rays and γ-Rays
11.4.3. Temperature Dependence of Decay Time and Efficiency of CsI(Tl) and "Pure" Csl
12.1.5. Scattered Radiation in γ-Ray Spectrometry
12.1.9. The Photo-fraction and γ-Ray Detection Efficiency
12.1.13. Total Absorption and Summing Spectrometers
12.1.14. Anti-coincidence Shielding
12.1.15. Sum-coincidence Spectrometry
12.2.5. Heavy Particle Spectrometry
12.2.7. Dual Scintillator Systems
13.1.6. Intermediate Energy Neutron Detection
13.4. Inorganic Glass Scintillators
13.5. Other Inorganic Scintillators
14.2. Inert Gases
14.2.10. Light Amplification by an Electric Field
14.3.2. Liquid Helium and Argon
References
Author Index
Volumes Published in the Series on Electronics and Instrumentation
- Edition: 1
- Published: January 1, 1964
- No. of pages (eBook): 684
- Imprint: Pergamon
- Language: English
- Hardback ISBN: 9780080104720
- Paperback ISBN: 9781483123646
- eBook ISBN: 9781483156064
Read The Theory and Practice of Scintillation Counting on ScienceDirect