LIMITED OFFER
Save 50% on book bundles
Immediately download your ebook while waiting for your print delivery. No promo code needed.
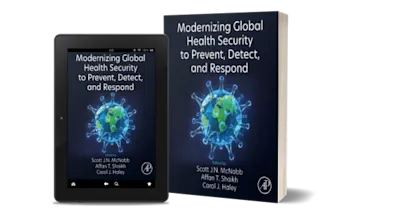
Smart Organ-on-Chip Devices: Dynamic Microfluidic Systems for Cell Culture discusses the concepts to engineer functional stimuli responsive organotypic-on-chip devices and its a… Read more
LIMITED OFFER
Immediately download your ebook while waiting for your print delivery. No promo code needed.
Smart Organ-on-Chip Devices: Dynamic Microfluidic Systems for Cell Culture discusses the concepts to engineer functional stimuli responsive organotypic-on-chip devices and its application in several fields, including drug development, disease modeling, personalized medicine, and tissue engineering. Groundbreaking studies are presented throughout the book sections to reinforce the importance of adding more reliable and robust in vitro platforms able to closely emulate the dynamism of human physiology.
The authors present new information regarding in silico studies of cell spheroids within microfluidic devices, as well as step-by-step guidance on key procedures. Written for researchers, practitioners and students using microfluidic devices as platforms, by well-respected scientists from both academia and industry.
TB
PB
LC