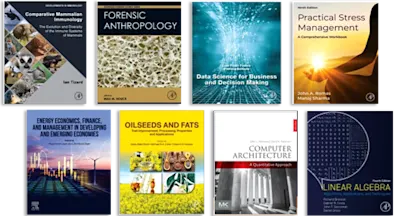
Severe Accidents in Sodium Fast Reactors
Safety Study Approach, Prevention and Mitigation by Design
- 1st Edition - November 26, 2024
- Imprint: Academic Press
- Editors: Frédéric Bertrand, Andrea Bachrata, Nathalie Seiler, Christophe Journeau
- Language: English
- Paperback ISBN:9 7 8 - 0 - 3 2 3 - 9 5 4 3 5 - 8
- eBook ISBN:9 7 8 - 0 - 3 2 3 - 9 5 4 3 6 - 5
Severe accidents in Sodium Fast Reactors: Safety Study Approach, Prevention and Mitigation by Design is a unique presentation of research work from the Sodium Fast Reactor Severe… Read more
Purchase options
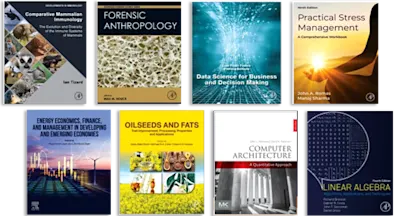
Severe accidents in Sodium Fast Reactors: Safety Study Approach, Prevention and Mitigation by Design is a unique presentation of research work from the Sodium Fast Reactor Severe Accident team at CEA. Focusing on in-core and out-core severe accident phenomena, this book analyzes severe accident scenarios and mitigation, related calculations and tools, and key outcomes from important experimental programs. The book describes the state-of-the-art on severe accident research which will help to inform and direct further pre-conceptual Generation IV SFRs. Lessons learned from the pre-conceptual phase of the French ASTRID project are highlighted alongside different approaches to demonstrate robustness.
To support the demonstration of concepts explored, a special section dedicated to calculation tools and their validation offers readers a unique set of tools to guide and inform their own research work. Readers will gain a deep understanding of methodologies to illustrate approaches as well as an overview of safety approaches from key countries based on the editor’s rich international collaboration.
To support the demonstration of concepts explored, a special section dedicated to calculation tools and their validation offers readers a unique set of tools to guide and inform their own research work. Readers will gain a deep understanding of methodologies to illustrate approaches as well as an overview of safety approaches from key countries based on the editor’s rich international collaboration.
- Written by a group of experts from CEA Cadarache on the research of the Sodium Fast Reactor and Severe Accidents Project
- Focuses on the safety and mitigation of future sodium reactor severe accidents
- Includes methodologies for the safety evaluation of SFRs to inform future designs
Nuclear engineers/researchers, reactor designers, academics and graduate students of generation IV and future reactors; safety experts and managers
1. Introduction
2. Dynamic and historical vision of severe accident approach in
SFR former projects
3. Overview of ASTRID and JSFR design
4. Severe accident scenario consideration and study methodology
for mitigation implementation by design
5. Severe accident calculation tools used for severe accident and
mitigation studies
6. Mitigation studies carried out for the French SFR
demonstrator
7. Experimental support studies for mitigation device design of
ASTRID
8. Japanese reactor mitigation approach and studies and
illustrations on other recent SFR concepts
9. Further R&D needs
10. Conclusion
2. Dynamic and historical vision of severe accident approach in
SFR former projects
3. Overview of ASTRID and JSFR design
4. Severe accident scenario consideration and study methodology
for mitigation implementation by design
5. Severe accident calculation tools used for severe accident and
mitigation studies
6. Mitigation studies carried out for the French SFR
demonstrator
7. Experimental support studies for mitigation device design of
ASTRID
8. Japanese reactor mitigation approach and studies and
illustrations on other recent SFR concepts
9. Further R&D needs
10. Conclusion
- Edition: 1
- Published: November 26, 2024
- Imprint: Academic Press
- Language: English
FB
Frédéric Bertrand
Frédéric Bertrand works in the French Alternative Energies, Atomic Energy Commission (CEA), France.
Affiliations and expertise
French Alternative Energies, Atomic Energy Commission (CEA), FranceAB
Andrea Bachrata
Andrea Bachrata works in the French Alternative Energies, Atomic Energy Commission (CEA), France.
Affiliations and expertise
French Alternative Energies, Atomic Energy Commission (CEA), FranceNS
Nathalie Seiler
Nathalie Marie works in the French Alternative Energies, Atomic Energy Commission (CEA), France.
Affiliations and expertise
French Alternative Energies, Atomic Energy Commission (CEA), FranceCJ
Christophe Journeau
Christophe Journeau works in the French Alternative Energies, Atomic Energy Commission (CEA), France.
Affiliations and expertise
French Alternative Energies, Atomic Energy Commission (CEA), FranceRead Severe Accidents in Sodium Fast Reactors on ScienceDirect