LIMITED OFFER
Save 50% on book bundles
Immediately download your ebook while waiting for your print delivery. No promo code needed.
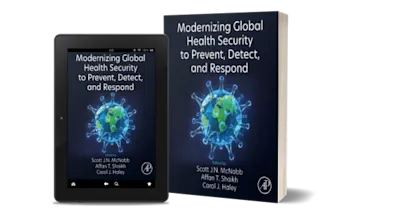
Sensors, Circuits, and Systems for Scientific Instruments: Fundamentals and Front-Ends presents a unified treatment of modern measurement systems by integrating relevant knowledge… Read more
LIMITED OFFER
Immediately download your ebook while waiting for your print delivery. No promo code needed.
Sensors, Circuits, and Systems for Scientific Instruments: Fundamentals and Front-Ends presents a unified treatment of modern measurement systems by integrating relevant knowledge in sensors, circuits, signal processing, and machine learning. It also presents detailed case studies of several real-life measurement systems to illustrate how theoretical analysis and high-level designs are translated into working scientific instruments.
The book is meant for upper-level undergraduate and beginning graduate students in electrical and computer engineering, applied physics, and biomedical engineering. It is designed to fill a gap in the market between books focused on specific components of measurement systems (semiconductor devices, analog circuits, digital signal processing, etc.) and books that provide a high-level "survey" or "handbook"-type overview of a wide range of sensors and measurement systems.
Senior-level undergraduates and beginning graduate students in electrical and computer engineering, applied physics, biomedical engineering, and related disciplines / Roughly 125 US schools offer an bioinstrumentation course taken by 9,000 students, while another 30 US schools offer an electronic instrumentation course. It is estimated that the global number is approximately double
SM