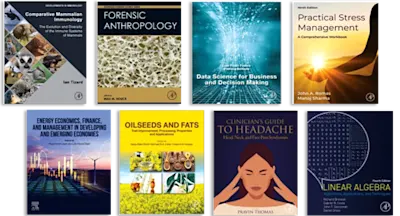
Scaling Up of Microbial Electrochemical Systems
From Reality to Scalability
- 1st Edition - January 28, 2022
- Imprint: Elsevier
- Editors: Dipak Ashok Jadhav, Soumya Pandit, S. Gajalakshmi, Maulin P. Shah
- Language: English
- Paperback ISBN:9 7 8 - 0 - 3 2 3 - 9 0 7 6 5 - 1
- eBook ISBN:9 7 8 - 0 - 3 2 3 - 9 0 7 6 6 - 8
Scaling Up of Microbial Electrochemical Systems: From Reality to Scalability is the first book of its kind to focus on scaling up of microbial electrochemical systems (MES) and… Read more
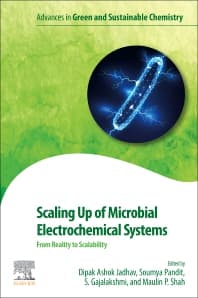
Purchase options
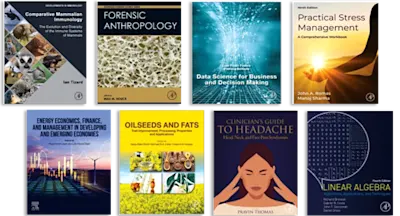
Institutional subscription on ScienceDirect
Request a sales quoteScaling Up of Microbial Electrochemical Systems: From Reality to Scalability is the first book of its kind to focus on scaling up of microbial electrochemical systems (MES) and the unique challenges faced when moving towards practical applications using this technology. This book emphasizes an understanding of the current limitations of MES technology and suggests a way forward towards onsite applications of MES for practical use. It includes the basics of MES as well as success stories and case studies of MES in the direction of practical applications.
This book will give a new direction to energy researchers, scientists and policymakers working on field applications of microbial electrochemical systems—microbial fuel cells, microbial electrolysis cells, microbial electrosynthesis cells, and more.
- Promotes the advancement of microbial electrochemical systems, from lab scale to field applications
- Illustrates the challenges of scaling up using successive case studies
- Provides the basics of MES technology to help deepen understanding of the subject
- Addresses lifecycle analysis of MES technology to allow comparison with other conventional methods
Bioelectrochemists, engineers, electrochemists, and chemists working on microbial electrochemical systems (MES), microbial fuel cells, environmental biotechnology, bioelectrochemical systems, bioenergy research and biological wastewater treatment; industries and academicians working on scaling up of bioelectrochemical systems, bioreactor designs and biological wastewater treatment. Bioenergy courses and elective courses offered for undergraduate and post graduate students; policymakers and NGOs
- Cover image
- Title page
- Table of Contents
- Copyright
- Contributors
- Chapter 1: Microbial electrochemical systems
- Abstract
- Acknowledgments
- 1.1: Introduction
- 1.2: Classification of METs
- 1.3: Conclusion
- Chapter 2: A review on scaling-up of microbial fuel cell: Challenges and opportunities
- Abstract
- 2.1: Introduction
- 2.2: MFC theory
- 2.3: Research gap of MFC
- 2.4: Operational and electrochemical limitations of MFC analysis
- 2.5: Technology development solution
- 2.6: Techno–economic viability
- 2.7: Pilot scale to industrial scale of MFC
- 2.8: Application of microbial fuel cell to the social relevance
- 2.9: Recent developments
- 2.10: Future improvements
- 2.11: Conclusion
- Chapter 3: Electroactive biofilm and electron transfer in microbial electrochemical systems
- Abstract
- Acknowledgments
- 3.1: Introduction
- 3.2: Electroactive microorganisms (EAMs)
- 3.3: Formation of electroactive biofilms (EABFs)
- 3.4: Electron transfer mechanism
- 3.5: Effect of design, operational, and biological parameters on electroactivity of EABFs
- 3.6: Genetic engineering: An approach to enhance exoelectrogenesis
- 3.7: Applications of EABFs
- 3.8: Conclusions and future prospects
- Chapter 4: Role of electroactive biofilms in governing the performance of microbial electrochemical system
- Abstract
- 4.1: Introduction
- 4.2: Role of electroactive biofilms in MES
- 4.3: Strategies for development of EAB
- 4.4: Microbes in EAB
- 4.5: Electron transfer in EAB
- 4.6: Methods to study EAB
- 4.7: Dynamic of EAB application
- 4.8: Conclusion and future prospects
- Chapter 5: Electroactive biofilm and electron transfer in the microbial electrochemical system
- Abstract
- 5.1: Introduction
- 5.2: Electroactive microorganism and biofilm formation
- 5.3: Factors affecting electroactive biofilm formation
- 5.4: Electron transfer mechanism in MES
- 5.5: Tools and techniques to study electroactive biofilms and microbial community analysis
- 5.6: Conclusion and future prospects
- Chapter 6: Electroactive biofilm and electron transfer in MES
- Abstract
- 6.1: Introduction
- 6.2: Electroactive biofilms (EABs)
- 6.3: Anodic electroactive biofilm
- 6.4: Cathodic electroactive biofilm
- 6.5: Mechanism of electron within anodic EAB
- 6.6: Mechanisms of electron transfer in cathodic EABs
- 6.7: Tools and techniques used to study EABs
- 6.8: Applications of EABs
- 6.9: Conclusion
- Chapter 7: Bioelectroremediation of wastes using bioelectrochemical system
- Abstract
- 7.1: Introduction
- 7.2: Drawbacks of conventional bioremediation
- 7.3: Phytoremediation
- 7.4: BES for ground water remediation
- 7.5: Practical obstacles in GW remediation suggests BES application
- 7.6: In situ bioelectroremediation: Ideal step
- 7.7: Bioelectroremediation: Future perspectives
- 7.8: Conclusion
- Chapter 8: Fiber-reinforced polymer (FRP) as proton exchange membrane (PEM) in single chambered microbial fuel cells (MFCs)
- Abstract
- 8.1: Introduction
- 8.2: Proton exchange membranes (PEM)
- 8.3: Present study
- 8.4: Designing and fabrication of single-chambered MFCs
- 8.5: Natural fiber-reinforced polymer (FRP) composite as PEM in MFCs
- 8.6: Substrates used in MFCs
- 8.7: Inocula used in MFCs
- 8.8: Experimental design
- 8.9: Results in terms of electricity generation
- 8.10: Results in terms of COD removal
- 8.11: Results of the comparison of different proton exchange membrane (PEM) used in MFC with commercially available PEM-based MFC
- 8.12: Results in terms of electricity generation
- 8.13: Results in terms of COD removal
- 8.14: Conclusions
- Chapter 9: Effects of biofouling on polymer electrolyte membranes in scaling-up of microbial electrochemical systems
- Abstract
- 9.1: Introduction
- 9.2: Causes of biofouling in polymer electrolyte membrane
- 9.3: Mechanism of polymer electrolyte membrane biofouling
- 9.4: Effects of biofouling on MES performance
- 9.5: Methods to analyze membrane biofouling
- 9.6: Challenges confronted in scaling-up of MES
- 9.7: Preventive measures of membrane biofouling
- 9.8: Conclusion
- Chapter 10: Advancement in electrode materials and membrane separators for scaling up of MES
- Abstract
- 10.1: Introduction
- 10.2: Designing of reactor to scale-up
- 10.3: Electrode modification in scaling-up of MES
- 10.4: Membrane separators in MES
- Chapter 11: Scale-up of bioelectrochemical systems: Stacking strategies and the road ahead
- Abstract
- 11.1: Introduction
- 11.2: Scale-up: Issues and strategies
- 11.3: Stacking of BESs
- 11.4: Voltage reversal and prevention
- 11.5: Pilot-scale BESs for hydrogen/methane production
- 11.6: Scaled-up BESs for bioremediation
- 11.7: Conclusions and future perspective
- Chapter 12: Application of microbial electrochemical system for industrial wastewater treatment
- Abstract
- 12.1: Introduction
- 12.2: Energy recovery in wastewater treatment systems
- 12.3: Industrial wastewater generation and the ecotoxicological impacts of the pollutants
- 12.4: Industrial wastewater treatment in microbial electrochemical systems
- 12.5: Recent advancements in scaling up microbial electrochemical systems
- 12.6: Economic and life cycle assessment
- 12.7: Conclusion
- Chapter 13: Metabolic engineering and synthetic biology key players for improving efficacy of microbial fuel cell technology
- Abstract
- Acknowledgment
- 13.1: Introduction
- 13.2: Classification or types and design of MFC for electricity generation
- 13.3: Molecular mechanisms of electron transfer by diverse microbial regimes or electrogens for MFC technology
- 13.4: Existing physical- and chemical-based approaches for improving the MFC performance
- 13.5: Existing pitfalls or drawbacks of existing MFC technology
- 13.6: Metabolic engineering and synthetic biology impacts on improving MFC performance
- 13.7: Conclusion and future outlook
- Chapter 14: Microbial electrochemical platform: A sustainable workhorse for improving wastewater treatment and desalination
- Abstract
- Acknowledgment
- 14.1: Introduction
- 14.2: Classification and general discussion about microbial electrochemical platform toward wastewater treatment and desalination
- 14.3: Potential role of existing native microbial regime in wastewater treatment and desalination
- 14.4: Metabolic engineering and synthetic biology impacts on improving strains or M/Os to improve the performance of MES/MFC toward wastewater treatment and desalination
- 14.5: Future outlook
- Chapter 15: Scaling-up of microbial electrochemical systems to convert energy from waste into power and biofuel
- Abstract
- 15.1: Introduction
- 15.2: Scale-up of MET from laboratory level to pilot level
- 15.3: Stacking of microbial electrochemical systems: A major perspective for scaling-up
- 15.4: Continuous mode of operation of microbial electrochemical systems during scale-up
- 15.5: Challenges for field-level application of MET
- 15.6: Solutions to overcome limitation of MET
- 15.7: Future perspective
- Chapter 16: Microbial fuel cell: Commercial aspect in terms of stacking and electrochemical perspective for scaling up
- Abstract
- 16.1: Introduction
- 16.2: Role of different materials in development of MFC
- 16.3: Strategies for development of MFC stacking
- 16.4: Modes of operation
- 16.5: MFC design for scale-up
- 16.6: Conclusion and future prospects
- Chapter 17: Scaling up and applications of microbial fuel cells
- Abstract
- 17.1: Introduction
- 17.2: Engineering design parameters for MFC scale-up
- 17.3: Process parameters for MFC scale-up
- 17.4: Criteria for scale-up
- 17.5: Market segmentation and SWOT analysis
- 17.6: Outlook
- Chapter 18: Electrode modification and its application in microbial electrolysis cell
- Abstract
- 18.1: Introduction
- 18.2: Electrode modification
- 18.3: MEC operation using modified electrodes
- 18.4: Limiting factors affecting the electrode function
- 18.5: Conclusions
- Chapter 19: Strategies in the direction of scaling-up aspects of microbial electrolysis cells
- Abstract
- 19.1: Introduction
- 19.2: Thermodynamic basics and theoretical advantages
- 19.3: Advantages of MECs over water electrolysis
- 19.4: MECs for methanation and microbial electrosynthesis
- 19.5: Engineering challenges that must be tackled
- 19.6: Interference of methanogens in H2 MECs
- 19.7: Strategies in the direction of scaling-up aspects of microbial electrolysis cells
- 19.8: Conclusions
- Chapter 20: Multi-stage constructed wetland microbial fuel cells: A new perspective for potential high-strength wastewater treatment under continuous operation
- Abstract
- 20.1: Introduction
- 20.2: Fundamentals of electron transfer mechanism and design of CW-MFCs
- 20.3: Potential high-strength wastewater treatment
- 20.4: Multi-stage systems
- 20.5: Advantages of multi-stage systems
- 20.6: Challenges in operation and future directions
- Chapter 21: Microbial fuel cells—Challenges for commercialization and how they can be addressed
- Abstract
- Acknowledgment
- 21.1: Introduction
- 21.2: Operating conditions and anodic constraints
- 21.3: Catholyte and cathodic conditions
- 21.4: Electrode materials
- 21.5: Proton exchange membrane
- 21.6: Oxygen reduction reaction catalysts
- 21.7: Design parameters and configurations
- 21.8: Scaling up and commercialization of MFC—Current status and challenges
- 21.9: Bottlenecks and future direction
- 21.10: Summary
- Chapter 22: Utilization of human waste and animal urine for energy and resource recovery in microbial electrochemical system
- Abstract
- 22.1: Introduction
- 22.2: Complexity of treatment of animal waste in a conventional wastewater treatment system and recovery options
- 22.3: Microbial electrochemical system (MES) for animal wastewater treatment
- 22.4: Animal waste treatment in MES
- 22.5: Mechanism and application of human waste and urine treatment in MES
- 22.6: Challenges and future perspectives for utilizing animal waste in MES
- 22.7: Conclusion
- Chapter 23: Application of artificial intelligence methods for the optimization and control of bioelectrochemical systems
- Abstract
- Acknowledgment
- 23.1: Introduction
- 23.2: Background
- 23.3: AI-based methods for studying BES
- 23.4: Conclusion and future outlook
- Chapter 24: Toward sustainable feasibility of microbial electrochemical systems to reality
- Abstract
- 24.1: Microbial electrochemical systems
- 24.2: Challenges
- 24.3: Energy efficiency
- 24.4: Economic assessment
- 24.5: Life cycle assessment (LCA)
- 24.6: Sustainable priorities
- 24.7: Conclusion and future outlook
- Index
- Edition: 1
- Published: January 28, 2022
- No. of pages (Paperback): 512
- No. of pages (eBook): 512
- Imprint: Elsevier
- Language: English
- Paperback ISBN: 9780323907651
- eBook ISBN: 9780323907668
DJ
Dipak Ashok Jadhav
Dipak Ashok Jadhav is a research professor at the Korea Maritime and Ocean University, Busan, South Korea. His research interests lie in microbial fuel cells, bioelectrochemical systems, sanitation, bioenergy research, water management, and waste-to-energy. He has received several prestigious awards and fellowship including Young Technological Innovation Award (GYTI), Young Engineers Award, Promising Engineers Award, Swachthon Award from Ministry, DAAD fellowship, Silver medal for post graduate studies at IIT Kharagpur, etc. His research area is microbial fuel cell, Bioelectrochemical system, Sanitation, Bioenergy research, Water management, Waste to Energy recovery. To his credits, there are over 33 papers in various peer-reviewed SCI journals including Renewable and Sustainable energy reviews, Bioresource Technology, Energy, Applied Biochemistry and Biotechnology, etc. and also authored 19 book chapters. He has presented the work at national and international conferences in the area of bioelectrochemical system and bioenergy. He is also serving as editorial board member /reviewers for International Journals and member for several scientific societies in the field of microbial fuel cell and bioenergy research. He is also editor for book on microbial electrochemical system.
SP
Soumya Pandit
SG
S. Gajalakshmi
MS
Maulin P. Shah
Dr. Maulin P. Shah is an active researcher and microbial biotechnologist with diverse research interest. His primary interest is the environment, the quality of our living resources and the ways that bacteria can help to manage and degrade toxic wastes and restore environmental health. Consequently, His work has been focused to assess the impact of industrial pollution on microbial diversity of wastewater following cultivation dependant and cultivation independent analysis.