LIMITED OFFER
Save 50% on book bundles
Immediately download your ebook while waiting for your print delivery. No promo code needed.
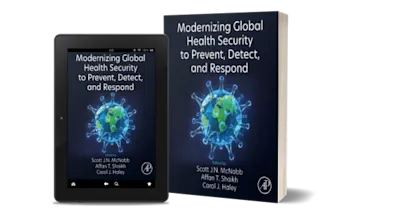
Rehabilitation of Metallic Structural Systems Using Fiber-Reinforced Polymer (FRP) Composites, Second Edition provides comprehensive knowledge on the application of FRPs in variou… Read more
LIMITED OFFER
Immediately download your ebook while waiting for your print delivery. No promo code needed.
Rehabilitation of Metallic Structural Systems Using Fiber-Reinforced Polymer (FRP) Composites, Second Edition provides comprehensive knowledge on the application of FRPs in various types of metallic field structures. Part I provides an overview of the various types of materials and systems and discusses the durability of bonds. Part II focuses on materials-level considerations, such as corrosion and mechanical behavior, putty effects on the effectiveness of pipeline systems, laser joining and the use of carbon and basalt FRP for underwater repair. Building on Part II, the final three sections focus on applications of FRP composites to steel components and various infrastructure systems.
This book will be a standard reference for civil engineers, designers, materials scientists, and other professionals who are involved in the rehabilitation of metallic structures using fiber reinforced polymer composites.
VK