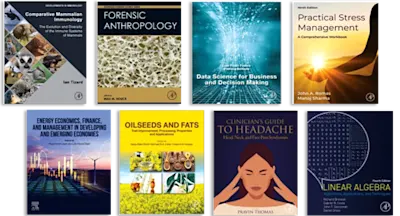
Rail Infrastructure Resilience
A Best-Practices Handbook
- 1st Edition - June 27, 2022
- Imprint: Woodhead Publishing
- Editors: Rui Calcada, Sakdirat Kaewunruen
- Language: English
- Paperback ISBN:9 7 8 - 0 - 1 2 - 8 2 1 0 4 2 - 0
- eBook ISBN:9 7 8 - 0 - 1 2 - 8 2 1 0 4 3 - 7
Economic growth, security and sustainability across Europe are at risk due to ageing railway infrastructure systems. At present, the majority of such systems are aging and some… Read more
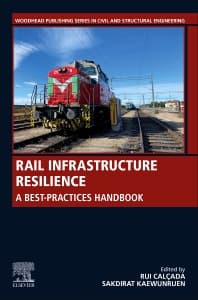
Purchase options
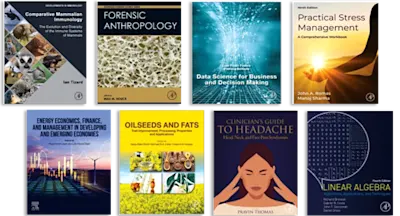
Institutional subscription on ScienceDirect
Request a sales quoteEconomic growth, security and sustainability across Europe are at risk due to ageing railway infrastructure systems. At present, the majority of such systems are aging and some have even reached their initial design lives. These issues align with a major challenge in civil engineering: how to restore and improve urban infrastructure and built environments. Policy, environmental and physical barriers must be addressed and overcome. The complex and interconnected nature of the problem means that there is a need for academia, industry, communities and governments to work collaboratively. The challenges posed by extreme events from natural and man-made disasters are urgent.
Rail Infrastructure Resilience: A Best-Practices Handbook presents developed improvement methods for rail infrastructure systems, toward resilience to extreme conditions. It shows how best to use new information in the engineering design, maintenance, construction and renewal of rail infrastructure resilience, through knowledge exchange and capability development. The book presents the outcome of a major European research project, known as the RISEN project. RISEN aimed to enhance knowledge creation and transfer using both international and intersectoral secondment mechanisms among European Advanced Rail Research Universities and SMEs, and Non-EU, leading rail universities, providing methodological approaches and practical tools for restoring and improving railway infrastructure systems for extreme events. Edited and written by members of this project, this book will be essential reading for researchers and practitioners hoping to find practical solutions to the challenges of rail infrastructure resilience.
Rail Infrastructure Resilience: A Best-Practices Handbook presents developed improvement methods for rail infrastructure systems, toward resilience to extreme conditions. It shows how best to use new information in the engineering design, maintenance, construction and renewal of rail infrastructure resilience, through knowledge exchange and capability development. The book presents the outcome of a major European research project, known as the RISEN project. RISEN aimed to enhance knowledge creation and transfer using both international and intersectoral secondment mechanisms among European Advanced Rail Research Universities and SMEs, and Non-EU, leading rail universities, providing methodological approaches and practical tools for restoring and improving railway infrastructure systems for extreme events. Edited and written by members of this project, this book will be essential reading for researchers and practitioners hoping to find practical solutions to the challenges of rail infrastructure resilience.
- Offers a best-practices handbook for rail infrastructure resilience from the leaders in the field
- Paints a holistic picture of the rail transport system, showing that infrastructure maintenance intervention can be enhanced through advanced monitoring systems and resilience design
- Presents rail infrastructure resilience and advanced condition monitoring, allowing a better understanding of the critical maintenance, renewal and retrofit needs of railways
- Considers how academia, industry, communities and governments can work collaboratively in order to tackle aggregated problems in rail infrastructure resilience
- Presents the findings from the RISEN project, the leading European project on enhancing knowledge creation and transfer of expertise on rail infrastructure resilience
Civil and railway engineers; Passenger rail and service providers; Infrastructure maintenance providers; Researchers and students of rail infrastructure and transportation systems.
Stakeholders in the resilience of railways; governments and civil authorities tasked with hardening critical infrastructure against extreme events; government agencies such as environment and transport.
Stakeholders in the resilience of railways; governments and civil authorities tasked with hardening critical infrastructure against extreme events; government agencies such as environment and transport.
- Cover image
- Title page
- Table of Contents
- Copyright
- Contributors
- About the Editors
- Foreword
- Acknowledgments
- 1: Introduction
- Abstract
- 1.1: Background
- 1.2: Railway infrastructure resilience
- 1.3: Advanced condition monitoring
- 1.4: Conclusions
- References
- 2: Railway vulnerability and resilience
- Abstract
- Acknowledgments
- 2.1: Railway system vulnerability and resilience analyses
- 2.2: Methodologies for railway vulnerability and resilience
- 2.3: Railway vulnerability and resilience practices in Chinese cities
- 2.4: Conclusions
- References
- 3: Rail resilience to climate change: Embedding climate adaptation within railway operations
- Abstract
- 3.1: Introduction
- 3.2: Best practice in climate adaptation and resilience
- 3.3: Rail Adapt Framework for climate change adaptation
- 3.4: Conclusions
- References
- 4: Rail transport resilience to demand shocks and COVID-19
- Abstract
- 4.1: Introduction
- 4.2: Rail transport demand shocks
- 4.3: Impacts of COVID-19 on rail demand and supply
- 4.4: Rail system resilience
- 4.5: Supply losses
- 4.6: Demand spikes
- 4.7: Demand losses
- 4.8: Increasing demand loss resilience
- 4.9: Concluding remarks
- References
- 5: Management of railway stations exposed to a terrorist threat
- Abstract
- 5.1: Introduction
- 5.2: Previous studies
- 5.3: Security risk analysis
- 5.4: Managing terrorism risk
- 5.5: The technology and terrorist threat
- 5.6: Emergency and preincident management
- 5.7: Conclusion
- References
- 6: Rail infrastructure systems and hazards
- Abstract
- 6.1: Extreme temperature
- 6.2: Earthquakes
- 6.3: Flooding
- 6.4: Summary
- References
- 7: Wheel-rail dynamic interaction
- Abstract
- 7.1: Introduction
- 7.2: Modeling of wheel-rail dynamic interaction
- 7.3: Detection and maintenance
- References
- 8: Wheel-rail interface under extreme conditions
- Abstract
- 8.1: Introduction into the wheel-rail interface
- 8.2: Basics of the wheel-rail contact
- 8.3: The wheel-rail interface under extreme conditions
- References
- 9: Train and track interactions
- Abstract
- Acknowledgments
- 9.1: Introduction: An overview of train and track interactions
- 9.2: Models of train and track interactions
- 9.3: Vehicle-track interaction due to differential subgrade settlement
- 9.4: Vehicle-track interaction due to polygonal wheel under traction condition
- 9.5: Vehicle-track interaction under extreme weather conditions
- 9.6: Conclusions
- References
- 10: Approaches for weigh-in-motion and wheel defect detection of railway vehicles
- Abstract
- Acknowledgments
- 10.1: Introduction
- 10.2: Propose approaches to obtain WIM and wheel defect detection
- 10.3: Numerical modeling
- 10.4: Results and discussion
- 10.5: Conclusion
- References
- 11: Railway ground-borne vibrations: Comprehensive field test development and experimental validation of prediction tools
- Abstract
- Acknowledgments
- 11.1: Introduction
- 11.2: Experimental characterization of the Carregado test site
- 11.3: Numerical modeling of ground-borne vibrations
- 11.4: Experimental validation
- 11.5: Conclusions
- References
- 12: Lateral resistance of different sleepers for the resilience of CWR tracks
- Abstract
- 12.1: Introduction
- 12.2: Fastening/sleeper resistance
- 12.3: The effect of ballast specifications on the lateral resistance
- 12.4: Influence of the sleeper type and shape on lateral resistance
- 12.5: Numerical assessment of sleeper lateral resistance
- 12.6: Ballast components contribution to lateral resistance for different sleepers
- 12.7: Conclusion
- References
- 13: Diagnostics and management methods for concrete sleepers
- Abstract
- 13.1: Sleeper design process
- 13.2: Prestressed concrete railway sleeper subject to dynamic load
- 13.3: Fatigue assessment for prestressed concrete sleeper
- 13.4: Rail seat abrasion
- 13.5: Time-dependent behavior of prestressed concrete sleepers
- 13.6: Summary
- References
- 14: Railway ballast
- Abstract
- 14.1: Introduction
- 14.2: Ballast degradation
- 14.3: Ballast inspection and assessment
- References
- 15: Railway turnouts and inspection technologies
- Abstract
- Acknowledgments
- 15.1: Introduction
- 15.2: Components of turnouts
- 15.3: Inspection
- 15.4: Maintenance
- 15.5: Lifecycle cost
- 15.6: Conclusions
- References
- 16: Risk-based maintenance of turnout systems
- Abstract
- 16.1: Introduction
- 16.2: Railway turnouts
- 16.3: Identification of a risk analysis method
- 16.4: Establishment of risk-based maintenance
- 16.5: Environmental impact consideration into a maintenance chain
- 16.6: Concluding remarks
- References
- 17: Railway bridge under increased traffic demands
- Abstract
- 17.1: Introduction
- 17.2: Monitoring program
- 17.3: Processing data
- 17.4: Analysis of results
- 17.5: Conclusion
- References
- 18: Structural health monitoring strategy for damage detection in railway bridges using traffic induced dynamic responses
- Abstract
- Acknowledgments
- 18.1: Introduction
- 18.2: Literature review on SHM for damage detection
- 18.3: Railway bridge over the Sado River
- 18.4: Strategy for damage detection using train induced dynamic responses
- 18.5: Conclusions
- References
- 19: Improved dynamic resilience of railway bridges using external dampers
- Abstract
- Acknowledgments
- 19.1: Dampers for structural vibration mitigation
- 19.2: Equation of motion for a bridge with viscous damper
- 19.3: Real-time hybrid simulation and testing
- 19.4: Response of the bridge-damper systems
- 19.5: Laboratory testing of an FVD
- 19.6: Comparison of damper performance
- 19.7: Conclusions
- References
- 20: Responses of mast structure and overhead line equipment (OHLE) subjected to extreme events
- Abstract
- 20.1: Introduction
- 20.2: Maintenance criteria
- 20.3: Vibration characteristics of OHLE
- 20.4: OHLE under harsh environment
- 20.5: Summary
- References
- 21: Reliability quantification of the overhead line conductor
- Abstract
- Acknowledgments
- 21.1: Introduction
- 21.2: Concept of reliability analysis
- 21.3: Load calculations
- 21.4: Results and discussions
- 21.5: Conclusions
- Data availability
- References
- Index
- Edition: 1
- Published: June 27, 2022
- No. of pages (Paperback): 494
- No. of pages (eBook): 494
- Imprint: Woodhead Publishing
- Language: English
- Paperback ISBN: 9780128210420
- eBook ISBN: 9780128210437
RC
Rui Calcada
Professor Rui Calcada is Full Professor in the Faculty of Engineering at the University of Porto, in Portugal, Head of the Civil Engineering Department, and Coordinator of the CSF-Centre of Competence in Railways. He is a Member of the Scientific Council of FEUP, and a Member of the Management Board of the R&D unit CONSTRUCT, in the Institute of R&D in Structures and Construction. He received his PhD and Habillation in civil engineering from the University of Porto. His research focusses include advanced models for analysis of the train-infrastructure dynamic interaction; dynamic effects on bridges and transition zones; fatigue assessment for railway bridges; track-structure interaction; condition monitoring systems; and advanced algorithms for condition assessment of railway infrastructure. He is the Principle Investigator of 12 major research projects. He currently works on the European Commission’s RISEN Project (Rail Infrastructure Systems Engineering Network), which has received funding from the EU’s Horizon 2020 program.
Affiliations and expertise
Professor, Civil Engineering Department and Coordinator of the Centre of Competence in Railways, Faculdade de Engenharia da Universidade do Porto, PortugalSK
Sakdirat Kaewunruen
Dr Sakdirat Kaewunruen is Senior Lecturer in Railway and Civil Engineering in the School of Civil Engineering at the University of Birmingham in the UK. He is also Coordinator of the RISEN project. He has extensive industry experience in the field of structural, civil and track engineering both in industry and academia. With over 14 years in the rail industry and regulatory environments prior to joining academia, he has an array of research interests, including rail engineering, track design, track components, structural and geotechnical engineering, maintenance and construction. He received his PhD in civil engineering from the University of Wollongong in Australia, and has also completed an Emerging Leader Program with the John F. Kennedy School of Government at Harvard University. He coordinates the EU-funded RISEN project and is a CI of S-CODE. He has lead numerous projects, sits on various industry committees, and has published widely in the field.
Affiliations and expertise
Senior Lecturer in Railway and Civil Engineering, School of Civil Engineering, Birmingham Centre for Railway Research and Education, Gisbert Kapp Building, University of Birmingham, Edgbaston, UKRead Rail Infrastructure Resilience on ScienceDirect