LIMITED OFFER
Save 50% on book bundles
Immediately download your ebook while waiting for your print delivery. No promo code needed.
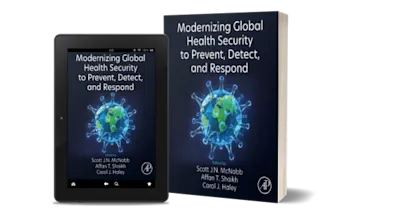
Proteomics, Multi-Omics and Systems Biology in Optic Nerve Regeneration is a comprehensive reference that covers all vistas of standardization of axon regeneration, as well as a… Read more
LIMITED OFFER
Immediately download your ebook while waiting for your print delivery. No promo code needed.
Proteomics, Multi-Omics and Systems Biology in Optic Nerve Regeneration is a comprehensive reference that covers all vistas of standardization of axon regeneration, as well as all multi-omics and system level data and integration tools. By adopting a translational approach, the book bridges current research in the field to clinical applications, and readers can expect to learn standardization approaches for axon regeneration, multi-omics datasets, different databases, search engines, multiple dataset integrative tools, pathway convergence approaches and tools, outcome and outcome measures that unify bench research with clinical outcome.
The axon regeneration from existing neurons in central nervous system (CNS) have become a potential possibility in the last decade. The potential possibility of long-distance axon growth has opened the possibility of re-connectivity of axons of retinal ganglion cell neurons within the lateral geniculate nucleus in the brain. The long-distance axon regeneration and re-connectivity is a promise to restore lost vision in the optic nerve. Further, long-distance regeneration and re-innervation is equally helpful for other fields such as spinal cord injuries.
Researchers engaged in neuro-regeneration including graduate students and ophthalmologists who are actively working in neuro-regeneration, glaucoma, traumatic optic neuropathies, bioinformaticians, systems biologists, proteomics, Basic scientists working on axon/dendrite regeneration, Clinicians, Ophthalmologists, Researchers in other neuro-degeneration fields (Alzeheimer’s diabetic peripheral neuropathy), Neuroscientists, Medical doctors who are working in the fields of Neuroscience, Scientists who are working in neuro-regeneration, researchers
SB
Sanjoy Bhattacharya is a tenured full Professor at the Department of Ophthalmology, University of Miami. He earned his doctorate in Biochemical Engineering and Biotechnology. Following postdoctoral research in epigenetics, including the publication of a “full” article in Nature on February 18,1999, and another in PNAS, USA on May 25, 1999, Dr. Bhattacharya utilized mass spectrometry to investigate the underlying mechanisms of enzyme activity associated with epigenetic alterations. He pursued the field of structural biology, namely X-crystallography, and continued to utilize mass spectrometry to investigate the mechanisms of protein crystallization imperfections.
Dr. Bhattacharya subsequently decided to embark on a career in vision research. In 2003, he initiated an independent research program in glaucoma and identified two protein molecules, Cochlin in the trabecular meshwork and Peptidyl arginine deiminase 2 in the optic nerve respectively. Sanjoy is one of the pioneering individuals who has brought power of unbiased proteomic, lipidomics and metabolomics isotopic ratio outlier analysis mass spectrometric approaches in in the field of ophthalmology and vision research. Currently, he is involved in characterizing the lipidome of tissues of the posterior eye and investigating the biological significance of lipid asymmetry in the cellular milieu. His laboratory, with its diverse expertise in engineering, computer programming, biology, and mass spectrometry, is dedicated to studying the complete set of molecules and biological systems involved in axon regeneration. He is collaborating with over 32 leading laboratories worldwide to comprehensively contribute multiomics data on axon regeneration in tissues (optic nerve and spinal cord), isolated neurons (retinal ganglion cells) and fractionated axons, mitochondria and tissue/cell secretome. His laboratory, which receives funding from federal agencies such as the National Institutes of Health and the Department of Defense, is seeking answers to fundamental questions as to what triggers expansion of biological membranes pertinent to axon regeneration. His group is also aiming to understand what biological roles the asymmetric lipid distribution plays for axon membrane expansion. He has helped create “axon regeneration” as a movement by students, trainees, physicians and scientists, aiming to expedite the delivery of novel therapeutics to patients within a decade. To support this endeavor, he helped create a non-profit organization Miami Metabolomics Research Support Group. He serves on the editorial board of several peer-reviewed journals including PLoS One as an academic editor, Experimental Eye Research as Special issues and Review editor, and Translational Metabolomics as the editor in chief. Sanjoy has held several leadership positions such as being chair of scientific advisory board of Malignancy Research Foundation, as a member of board of trustees of Association of Ocular Pharmacology and Therapeutics and as Chair of Miller School of Medicine Faculty Council. He is inaugural director of a trailblazing master’s program in Vision Science and Investigative Ophthalmology. He is also the founding director of Miami Integrative Metabolomics Research Center. His laboratory is well-reputed for mentorship of students. A significant number of his former postdoctoral fellows and graduate students currently occupy faculty professor positions or hold prominent senior scientist roles in industry.