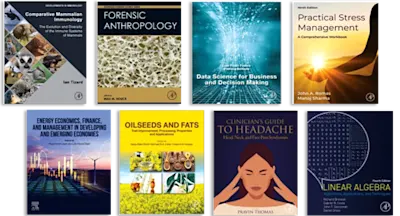
Progress and Recent Trends in Microbial Fuel Cells
- 1st Edition - June 7, 2018
- Imprint: Elsevier
- Authors: Patit Paban Kundu, Kingshuk Dutta
- Language: English
- Paperback ISBN:9 7 8 - 0 - 4 4 4 - 6 4 0 1 7 - 8
- eBook ISBN:9 7 8 - 0 - 4 4 4 - 6 4 0 1 8 - 5
Progress and Recent Trends in Microbial Fuel Cells provides an in-depth analysis of the fundamentals, working principles, applications and advancements (including co… Read more
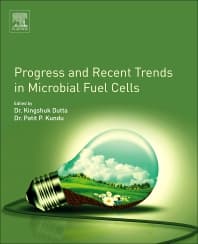
Purchase options
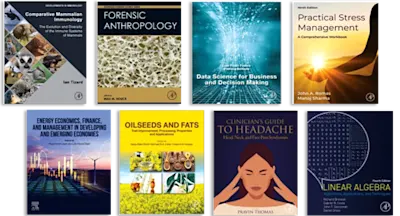
Institutional subscription on ScienceDirect
Request a sales quoteProgress and Recent Trends in Microbial Fuel Cells provides an in-depth analysis of the fundamentals, working principles, applications and advancements (including commercialization aspects) made in the field of Microbial Fuel Cells research, with critical analyses and opinions from experts around the world. Microbial Fuel cell, as a potential alternative energy harnessing device, has been progressing steadily towards fruitful commercialization. Involvements of electrolyte membranes and catalysts have been two of the most critical factors toward achieving this progress. Added applications of MFCs in areas of bio-hydrogen production and wastewater treatment have made this technology extremely attractive and important.
.
- Reviews and compares MFCs with other alternative energy harnessing devices, particularly in comparison to other fuel cells
- Analyses developments of electrolyte membranes, electrodes, catalysts and biocatalysts as critical components of MFCs, responsible for their present and future progress
- Includes commercial aspects of MFCs in terms of (i) generation of electricity, (ii) microbial electrolysis cell, (iii) microbial desalination cell, and (iv) wastewater and sludge treatment
Chemists and biochemical engineering students and researchers in alternative energy, fuel cells, microbial fuel cells, membranes, waste-water treatment and bio-hydrogen
Chapter 1: Introduction to microbial fuel cells
1.1. Background and significance
1.2. Working principle
1.3. Components and features
1.4. Technologies based on MFCs
1.5. Future expectations from MFCs
References
Author(s): Kingshuk Dutta and Patit P. Kundu
Chapter 2: Performance trends and status of microbial fuel cells
2.1. Introduction
2.2. Comparison with hydrogen fuel cells
2.3. Comparison with direct alcohol fuel cells
2.4. Comparison with passive alcohol fuel cells
2.5. Comparison with solid oxide fuel cells
2.6. Comparison with molten carbonate fuel cells
2.7. Comparison with alkaline fuel cells
2.8. Comparison with phosphoric acid fuel cells
2.9. Comparison with existing battery technologies and alternative energy resources
2.10. Further information
2.11. Relevance and outlook
References
Author(s): Vikash Kumar, Ruchira Rudra, Subrata Hait, Piyush Kumar and Patit P. Kundu
Chapter 3: Configurations of microbial fuel cells
3.1. Introduction
3.2. Normal configuration and general requirements
3.2.1. Uncoupled bioreactor MFC
3.2.2. Integrated bioreactor MFC
3.2.3. MFC with direct electron transfer
3.2.4. MFC with mediated electron transport
3.3. General requirements
3.3.1. Anode
3.3.2. Cathode
3.3.3. Membranes
3.4. Easy to build fuel cell configurations
3.4.1. Dual chamber H-type MFC
3.4.2. Dual chamber MFC
3.4.3. Dual chamber MFC with water soluble catholytes
3.4.4. Simple air cathode MFC
3.4.4.1. Cube type MFC
3.4.4.2. Cylindrical air cathode MFC
3.5. Innovative designs
3.5.1. Flat plate MFC
3.5.2. Biosolar MFC
3.5.3. Tubular packed bed MFC for continuous operation
3.5.4. Stacked MFC
3.5.5. Membraneless MFC
3.5.6. Biocathode MFC
3.5.7. Origami star inspired fuel cell
3.5.8. 3D paper based MFC
3.6. Reactors design and efficiency
3.7. Operation and assessment
3.8. Applications
3.9. Future directions
3.10. Conclusion
References
Author(s): Arpita Nandy and Patit P. Kundu
Chapter 4: Polymer electrolyte membranes for microbial fuel cells: Part A. Nafion-based membranes
4.1. Introduction
4.2. Functions of the PEM in MFC
4.3. Property requirements of the membrane materials
4.4. Fluorinated membrane structure required for efficient MFC operation
4.5. Present research on Nafion-based membranes
4.5.1. Nafion blends and composites
4.5.2. Nafion/fluorinated polymers
4.5.3. Others PEMs
4.6. Membrane characterizations
4.6.1. Structural characterizations
4.6.1.1. X-ray diffraction
4.6.1.2. Imaging techniques: Scanning electron and transmission electron microscopies
4.6.1.3. Porosity
4.6.2. Ion exchange capacity
4.6.3. Proton conductivity
4.6.4. Mechanical characterizations
4.7. Performance evaluations
4.8. Existing challenges of PEM technology
4.8.1. Ohmic resistance
4.8.2. Oxygen diffusion
4.8.3. Substrate crossover
4.8.4. Biofouling
4.9. Future directions
References
Author(s): Piyush Kumar, Ram P. Bharti, Vikash Kumar and Patit P. Kundu
Chapter 5: Polymer electrolyte membranes for microbial fuel cells: Part B. Non-nafion alternative membranes
5.1. Introduction
5.2. Present research of non-Nafion-based membranes
5.3. Conclusion, existing challenges and future perspectives
References
Author(s): Suparna Das, Kingshuk Dutta, Swapan K. Bhattacharya and Patit P. Kundu
Chapter 6: Bipolar membranes for microbial fuel cells
6.1. Introduction: Definition and general description of the use of bipolar membranes in microbial fuel cells
6.2. Preparation and application of bipolar membranes in MFCs
6.3. Conclusion, existing challenges and future perspectives
References
Author(s): Kingshuk Dutta and Patit P. Kundu, Prasenjit Bhumia
Chapter 7: Low-cost solutions for fabrication of microbial fuel cells: Ceramic separator and electrode modifications
7.1. Introduction
7.2. Fundamentals of MFCs and its components
7.2.1. Anode materials
7.2.1.1. Anode modification using conductive polymers
7.2.1.2. Anode modification using graphene and CNT
7.2.1.3. Anode modification using metal oxides
7.2.1.4. Anode modification by electrochemical oxidation
7.2.2. Cathode materials
7.2.3. Current collectors
7.2.4. Separators
7.3. Properties of clay used in ceramic separators
7.3.1. Mechanism of cation exchange through clay
7.3.2. Strengthening the clay based separators
7.3.3. Modification in the clay mineral composition to enhance cation exchange
7.3.4. Ceramic separator as low-cost solution for electrochemical devices
7.3.5. Performance of MFCs with ceramic separator
7.4. Importance of ORR catalysts and related mechanism: Options of low-cost cathode catalysts
7.4.1. Non-metal and metal impregnated carbon catalysts
7.4.2. Transition metal oxides
7.4.3. Metal doped complex organic catalysts
7.4.4. Cost analysis of catalysts
7.5. Scalable MFCs and stacking
7.6. Concluding remarks
References
Author(s): Md. T. Noori, Pritha Chatterjee, M. M. Ghangrekar and C. K. Mukherjee
Chapter 8: Electrodes for microbial fuel cells
8.1. Introduction
8.2. Electrode materials and their desired properties
8.2.1. Conductivity
8.2.2. Durability and stability
8.2.3. Porosity and surface area
8.2.4. Biocompatible nature
8.2.5. Cost and availability
8.3. Electrode material types
8.3.1. Carbon-based electrode materials
8.3.2. Metal electrodes
8.3.3. Composite electrode materials
8.4. Surface modification of electrodes
8.4.1. Modification with metals or metal oxides
8.4.2. Modification with polymers
8.4.3. Modification with composite materials
8.5. Electrode cost
8.6. Existing challenges and future perspective
References
Author(s): Usha Kumari, Ravi Shankar and Prasenjit Mondal
Chapter 9: Anode catalysts and biocatalysts for microbial fuel cells
9.1. Introduction
9.2. Functions of the catalysts
9.3. Property requirements of the catalysts
9.4. Present research
9.4.1. Materials of electrocatalysts
9.4.1.1. Carbonaceous anode based materials
9.4.1.2. Metal based materials
9.4.1.3. Conducting polymers
9.4.2. Microbes
9.4.2.1. Bacterial species used as MFC biocatalyst
9.4.2.2. Yeast in MFC
9.4.2.3. Mixed community
9.5. Catalyst characterizations
9.5.1. 16S rRNA
9.5.2. DGGE
9.5.3. FISH
9.5.4. RFLP, SSCP and ARISA
9.5.5. qRT-PCR
9.5.6. GS-FLX
9.5.7. QCM
9.5.8. FAME
9.6. Performance evaluations
9.6.1. Anode potential
9.6.2. Cyclic voltammetry
9.6.3. Electrochemical impedance spectroscopy (EIS)
9.7. Conclusion
References
Author(s): Yuan Li, Pier-Luc Tremblay and Tian Zhang
Chapter 10: Propellants of microbial fuel cells
10.1. Introduction
10.2. Nutrient requirements of MFC microorganisms
10.3. General characteristics of different fuels
10.3.1. Simple or defined substrates
10.3.1.1. Glucose
10.3.1.2. Acetate
10.3.1.3. Fructose
10.3.1.4. Sucrose
10.3.2. Complex defined substrates
10.3.2.1. Starch
10.3.2.2. Cellulose
10.3.2.3. Others
10.3.3. Complex undefined substrates
10.3.3.1. Activated sludge and algal biomass
10.3.3.2. Agro industrial wastewater
10.3.3.3. Brewery industry wastewater
10.3.3.4. Dairy industry wastewater
10.3.3.5. Domestic and municipal wastewater
10.3.3.6. Food processing industry wastewater
10.3.3.7. Livestock industry wastewater
10.3.3.8. Mining industry wastewater
10.3.3.9. Paper plant wastewater
10.3.3.10. Petrochemical industry wastewater
10.3.3.11. Pharmaceutical industry wastewater
10.3.3.12. Refinery and distillery industry waste water
10.3.3.13. Textile industry wastewater
10.4. Mechanism of fuel oxidation in MFC
10.5. Comparison of the efficiency of different fuels
10.6. Future aspects
References
Author(s): Anwesha Mukherjee, Rushika Patel and Nasreen S. Munshi
Chapter 11: Exoelectrogens for microbial fuel cells
11.1. Introduction
11.2. Mechanisms of electron transfer
11.2.1. Mediated electron transfer
11.2.1.1. Endogenous electron shuttles
11.2.1.2. Artificial electron shuttles
11.2.1.3. Primary metabolites
11.2.1.4. MET mechanisms for biofilms at the cathode
11.2.2. Direct electron transfer
11.2.2.1. G. sulfurreducens: OMC pathway
11.2.2.2. S. oneidensis: Mtr-pathway
11.2.2.3. DET in other organisms
11.2.2.4. DET at the cathode
11.2.3. Nanowires
11.3. Studies using known exoelectrogenic strains
11.4. Tools for studying exoelectrogens
11.4.1. Electrochemical Analysis
11.4.2. Microscopy
11.4.3. Biological analysis
11.4.4. Raman spectroscopy
11.5. Operational conditions
11.6. Future directions
11.7. Sources of further information
References
Author(s): Jeff R. Beegle and Abhijeet P. Borole
Chapter 12: Biofilm formation within microbial fuel cells
12.1. Introduction
12.2. Mechanism of biofilm formation
12.3. Electroactive biofilms
12.3.1. Challenges of electroactive biofilms
12.3.2. Factors affecting electroactive biofilm formation
12.3.2.1. System configuration
12.3.2.2. Operating conditions
12.3.2.3. Biological parameters
12.4. Conclusion and future directions
12.5. Sources of further information
References
Author(s): Ramya Veerubhotla, Jhansi L Varanasi and Debabrata Das
Chapter 13: Genetic approaches for improving performance of microbial fuel cells: Part A
13.1. Introduction
13.2. Electron transfer in life
13.3. Discovery of genes involved in electron transfer in MFCs
13.4. Metabolic pathways employed in MFC systems
13.4.1. Geobacter spp.
13.4.1.1. General features
13.4.1.2. Procedures assayed and results
13.4.1.3. Future possibilities
13.4.2. Shewanella spp.
13.4.2.1. General features
13.4.2.2. Procedures assayed and results
13.4.2.3. Future possibilities
13.4.3. Other heterotrophic microorganisms
13.4.3.1. General features
13.4.3.2. Procedures assayed and results
13.4.3.3. Future possibilities
13.5. Other metabolic pathways used in MFC systems
13.5.1. Chemolithoautotrophic metabolism
13.5.2. Photoautotrophic metabolism
13.6. Naturally assembled microbial communities to improve MFC performance
13.7. Artificially assembled anodic communities to improve MFC performance
13.8. Future directions
13.9. Sources of further information
References
Author(s): M. J. González-Pabón, F. Figueredo, E. L. M. Figuerola, A. Saavedra, L. Erijman and E. Cortón
Chapter 14: Genetic approaches for improving performance of microbial fuel cells: Part B
14.1. Introduction
14.2. Substrate processing and accessibility
14.2.1. Directed evolution of redox enzymes
14.2.2. Surface-display systems
14.2.2.1. Bacterial surface-display
14.2.2.2. Yeast surface-display
14.2.3. Bioremediation of contaminated soil and water
14.3. Improvement of electron transfer
14.3.1. Internal electron transfer
14.3.2. External electron transfer
14.4. Metabolic engineering
14.5. Enzyme and protein engineering
14.5.1. Protein immobilization
14.5.2. Engineered pilin
14.6. Concluding remarks
References
Author(s): Orr Schlesinger and Lital Alfonta
Chapter 15: Kinetics and mass transfer within microbial fuel cells
15.1. Introduction
15.2. Modeling approaches for MFCs
15.3. Case study - 1D analytical model for continuous operation
15.3.1. Model structure and flux balance
15.3.2. Model assumptions
15.3.3. Governing equation and boundary conditions
15.3.3.1. Mass transfer
15.3.3.2. Kinetics – Anode and cathode
15.4. Adaptation for batch operation
15.5. Modifications for a single chamber configuration
15.6. Summary
Nomenclature
References
Author(s): V. B. Oliveira, J. Vilas Boas and A. M. F. R. Pinto
Chapter 16: Biochemistry and electrochemistry at the electrodes of microbial fuel cells
16.1. Introduction
16.2. Biochemistry and electrochemistry at the electrodes
16.2.1. Underlying catabolic pathways for energy generation from microorganisms
16.2.2. Distinguished electron transport mechanism
16.2.2.1. Direct electron transport
16.2.2.2. Electron transport through mediators
16.2.2.3. Electron transport through conductive nanowires
16.2.3. Proton transport mechanism in MFCs
16.2.3.1. Cation exchange membrane (CEM)
16.2.3.2. Anion exchange membrane (AEM)
16.2.3.3. Bipolar membrane (BPM)
16.3. Underlying factors that affect the MFC performance
16.3.1. Ohmic losses
16.3.2. Activation losses
16.3.3. Bacterial metabolic losses
16.3.4. Concentration losses
16.4. Anode-microbe interactions
16.5. Summary
References
Author(s): Prasenjit Bhunia, Kingshuk Dutta
Chapter 17: Wastewater biorefinery based on microbial electrolysis cell: Opportunities and challenges
17.1. Introduction
17.1.1. Global energy and water security
17.1.2. Wastewater-based biorefinery
17.1.3. Microbial electrolysis cell (MEC)
17.1.4. H2 as fuel
17.1.5. Aim of the chapter
17.2. Bioelectrochemical system (BES)
17.2.1. History of BES
17.2.2. Types of BES
17.2.2.1. MFC
17.2.2.2. MEC
17.2.3. MEC systems and materials used for H2 production
17.2.3.1. Cathode and anode
17.2.3.2. MEC membranes
17.2.3.3. MEC system for tubing and gas collection
17.3. MEC configurations and factors affecting H2 production
17.3.1. Double-chambered MEC systems
17.3.1.1. High-performance double-chambered MEC
17.3.1.2. Bio-electrochemically assisted microbial reactor (BEAMR)
17.3.1.3. Concentric tubular double-chambered MEC
17.3.1.4. Enriched MEC bio-cathodes using sediment MFC bio-anodes
17.3.2. Single-chambered MEC systems
17.3.2.1. Single-chambered MEC with a flat carbon cathode and brush anode
17.3.2.2. Cathode on top single-chambered MEC
17.3.2.3. Up-flow single-chambered MEC
17.3.2.4. Bottle-type single-chambered MEC
17.3.3. Factors affecting production of H2 in MEC systems
17.3.3.1. pH
17.3.3.2. Temperature
17.3.3.3. Catalyst
17.3.3.4. Conductivity of solution
17.4. Thermodynamics of H2 production and MEC performance
17.4.1. H2 production and measurement in MEC
17.4.2. H2 yield of MEC
17.4.3. Energy yield of MEC
17.5. Challenges and opportunities in MEC technology
17.5.1. Energy losses in MEC system
17.5.1.1. Activation losses in MEC system
17.5.1.2. Coulombic losses in MEC system
17.5.1.3. Concentration losses in MEC system
17.5.2. Methanogenesis in MEC
17.5.3. Economics of MEC
17.5.4. Future outlooks of MEC
17.5.4.1. Technological approach
17.5.4.2. Methanogenesis inhibition
17.5.4.3. Pure culture versus mixed consortia studies
17.5.4.4. Electrode selection
17.6. Conclusions
References
Author(s): M. Waqas, M. Rehan, A. S. Aburiazaiza and A. S. Nizami
Chapter 18: Microbial fuel cells as a platform technology for sustainable wastewater treatment
18.1. Introduction
18.2. Wastewater treatment and energy needs
18.2.1. General overview of wastewater treatment
18.2.2. Energy consumption in wastewater treatment
18.3. Opportunities for energy recovery and savings in wastewater treatment
18.3.1. Hydraulic energy recovery
18.3.2. Heat recovery
18.3.3. Combined heat and power (CHP) systems
18.3.4. Biogas generation (anaerobic digestion, AD)
18.3.5. Microalgae growth for biofuels
18.3.6. Anammox process (novel configurations)
18.4. MFCs - Efficiency evaluations
18.4.1. Carbon removal
18.4.2. Nutrient removal
18.4.3. Energy efficiency
18.4.3.1. Estimated energy benefits
18.4.3.2. Comparison with aeration systems
18.4.3.3. Normalized energy recovery concept
18.4.3.4. Energy consumption in MFCs
18.4.3.5. Energy payback time
18.5. Existing challenges
18.5.1. Microbial kinetics
18.5.2. Electron acceptors
18.5.3. Electrode materials
18.5.4. Understanding of power density (process reliability and stability)
18.5.5. Other factors
18.6. Future directions
18.6.1. Process development
18.6.2. Resource recovery options
18.6.3. Large scale development
18.6.4. Integrated processes
18.6.4.1. Integrating with membrane processes
18.6.4.2. Integrating with aeration tank in conventional wastewater treatment plant
18.6.4.3. Integration with other bioelectrochemical systems
18.6.5. Biorefinery configurations
18.7. Summary
References
Author(s): Veera G. Gude
Chapter 19: Microbial desalination cell technology: Functions and future prospects
19.1. Introduction
19.1.1. Water-energy crisis in desalination
19.1.2. Microbial desalination cell – harvester of chemical energy
19.2. Essential concepts of microbial desalination cell
19.2.1. Operative principle
19.2.2. Performance factors: Analyses and calculations
19.2.3. Microbial desalination cells configurations
19.2.3.1. Air-cathode microbial desalination cell
19.2.3.2. Biocathode microbial desalination cell
19.2.3.3. Stacked microbial desalination cell
19.2.3.4. Recirculation microbial desalination cell
19.2.3.5. Microbial electrolysis desalination cell
19.2.3.6. Capacitive microbial desalination cell
19.2.3.7. Upflow microbial desalination cell
19.2.3.8. Osmotic microbial desalination cell
19.2.3.9. Bipolar membrane microbial desalination cell
19.2.3.10. Decoupled microbial desalination cell
19.2.3.11. Ion-exchange resin coupled microbial desalination cell
19.2.3.12. Five-chambered biocathode microbial desalination cell
19.2.3.13. Modularized filtration air cathode microbial desalination cell
19.3. Materials used in microbial desalination cells
19.3.1. Exoelectrogens
19.3.2. Substrates
19.4. Performance and efficiency of microbial desalination cell
19.4.1. Polarization and power density
19.4.2. COD removal efficiency
19.4.3. Electrochemical impedance spectroscopy
19.4.4. Cell potential (emf), concentration gradient and water transport
19.4.5. pH and electrolyte conductivity
19.4.6. External and internal resistance
19.4.7. Hydraulic retention time
19.5. Functional applications and scaleup
19.5.1. Wastewater treatment and water desalination
19.5.2. Water softening and metal ions removal
19.5.3. Groundwater remediation
19.6. Challenges to MDC technologies
19.7. Conclusions
References
Author(s): Carmalin Sophia A. and Jaydevsinh M. Gohil
Chapter 20: Coupled systems based on microbial fuel cells
20.1. Introduction
20.2. MFC-coupled wastewater treatment and the potential of MFC-MBRs
20.3. MFC-complemented anaerobic digestion
20.4. Conclusions
References
Author(s): Nándor Nemestóthy
Chapter 21: Commercialization aspects of microbial fuel cells
21.1. Introduction
21.2. Potentials of MFCs for commercialization
21.3. Prospective sector(s) for MFC applications
21.3.1. Wastewater treatment
21.3.2. Powering low energy devices
21.3.3. Robotics
21.4. Global status of MFCs commercialization / market leaders in MFCs
21.5. Current research towards commercialization
21.6. Challenges towards fruitful commercialization (lab to market bottleneck)
21.7. Future predictions and directions
References
- Edition: 1
- Published: June 7, 2018
- Imprint: Elsevier
- No. of pages: 464
- Language: English
- Paperback ISBN: 9780444640178
- eBook ISBN: 9780444640185
PK
Patit Paban Kundu
KD