Photovoltaics for Space
Key Issues, Missions and Alternative Technologies
- 1st Edition - October 26, 2022
- Editors: Sheila G. Bailey, Aloysius F. Hepp, Dale Ferguson, Ryne P. Raffaelle, Steven M. Durbin
- Language: English
- Paperback ISBN:9 7 8 - 0 - 1 2 - 8 2 3 3 0 0 - 9
- eBook ISBN:9 7 8 - 0 - 1 2 - 8 2 3 3 0 1 - 6
PV has traditionally been used for electric power in space. Solar panels on spacecraft are usually the sole source of power to run the sensors, active heating and cooling, and co… Read more
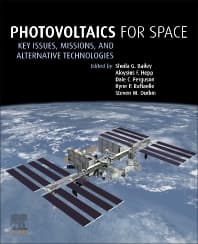
Purchase options
Institutional subscription on ScienceDirect
Request a sales quotePV has traditionally been used for electric power in space. Solar panels on spacecraft are usually the sole source of power to run the sensors, active heating and cooling, and communications. Photovoltaics for Space: Key Issues, Missions and Alternative Technologies provides an overview of the challenges to efficiently produce solar power in near-Earth space and beyond: the materials and device architectures that have been developed to surmount these environmental and mission-specific barriers. The book is organized in four sections consisting of detailed introductory and background content as well as a collection of in-depth space environment, materials processing, technology, and mission overviews by international experts. This book will detail how to design and optimize a space power system’s performance for power-to-weight ratio, effectiveness at end of operational life (EOL) compared to beginning of operational life (BOL), and specific mission objectives and goals.
This book outlines the knowledge required for practitioners and advanced students interested in learning about the background, materials, devices, environmental challenges, missions, and future for photovoltaics for space exploration.
- Provides an update to state-of-the-art and emerging solar cell technologies
- Features comprehensive coverage of solar cells for space exploration and materials/device technology options available
- Explains the extreme conditions and mission challenges to overcome when using photovoltaics in space
PART ONE Introduction: technologies, issues, and applications
1. An introduction to space photovoltaics: technologies, issues, and missions
Ryne P. Raffaelle
1.1 Introduction to the photovoltaic effect and solar cell
1.2 First-generation space photovoltaics: missions, technologies, and issues
1.3 The next era in space: new materials and missions in low-earth orbit
1.4 New device and advanced materials technologies into the 21st century
1.5 Exploration of Mars and beyond: notable solar-powered spacecraft
1.6 Conclusions
2. Space solar arrays and spacecraft charging
D.C. Ferguson, D.P. Engelhart, R.C. Hoffmann, V.J. Murray and E.A. Plis
2.1 Introduction to spacecraft charging
2.2 Arcing: effects, standards, and mitigation
2.3 Models of environment and charging
2.4 Conclusions: the necessity for testing
3. Air mass zero (AM0) studies and solar cell calibration
Carsten Baur, Emilio Fernández Lisbona and José Ramón González
3.1 Introduction
3.2 Primary calibration
3.3 Secondary calibration and measurements of MJ solar cells
3.4 Conclusions
4. Space applications of III-V single- and multijunction solar cells
Philip T. Chiu
4.1 Space solar cells: applications and challenges
4.2 Physics of single-junction solar cells
4.3 Silicon and gallium arsenide-based single-junction solar cells
4.4 Physics of multijunction solar cells
4.5 Indium gallium phosphide/gallium arsenide-based dual-junction solar cells
4.6 InGaP/GaAs/Ge-based 3J solar cells
4.7 Lattice-mismatched 3J solar cells
4.8 Lattice-mismatched quadruple (four)-junction solar cells
4.9 Lattice mismatched quintuple (five)-junction solar cells
4.10 Conclusions
5. Perovskite solar cells: background and prospects for space
power applications
Lyndsey McMillon-Brown and Timothy J. Peshek
5.1 Introduction
5.2 Characteristics of perovskite solar cells
5.3 Use of perovskites for space power: issues and opportunities for improvement
5.4 Conclusion
6. Photovoltaics and nuclear energy conversion for space power: background and issues
Mark Antonio Prelas, Tariq Rizvi Alam and Modeste Tchakoua Tchouaso
6.1 Introduction
6.2 Radiation damage
6.3 Radioisotopes
6.4 Energy conversion technologies
6.5 Conclusions
PART TWO Materials: focus on new technologies and advanced processing
7. Perovskite solar cells on the horizon for space power systems
Brandon K. Durant, Ian R. Sellers and Bibhudutta Rout
7.1 Background of perovskite solar cells
7.2 Defect tolerance and ion mobility
7.3 Particle radiation tolerance
7.4 Thermal stability
7.5 Conclusion and outlook
8. Thermophotovoltaic energy conversion in space
Alejandro Datas and Donald L. Chubb
8.1 Introduction
8.2 Thermal-to-electric energy conversion in space
8.3 Overview of thermophotovoltaic energy conversion
8.4 Thermophotovoltaic systems for space applications
8.5 Conclusions
9. Thin-film materials for space power applications
Ina T. Martin, Kyle Crowley and Aloysius F. Hepp
9.1 Introduction
9.2 Materials, devices, and impact of the space environment
9.3 Thin-film solar cells in space: past, present, and future
9.4 Conclusions
10. Inverted lattice-matched GaInP/GaAs/GaInNAsSb triple-junction solar cells: epitaxial lift-off thin-film devices and potential space applications
Naoya Miyashita and Yoshitaka Okada
10.1 Introduction
10.2 Design and growth of GaInNAs 1.0-eV subcells
10.3 Hybrid growth approach for MJSCs and ELO devices
10.4 Conclusion
11. Summary of the design principles of betavoltaics and space applications
Tariq Rizvi Alam, Modeste Tchakoua Tchouaso and Mark Antonio Prelas
11.1 Nuclear batteries
11.2 Different types of nuclear batteries
11.3 Betavoltaic batteries
11.4 Basic operation of betavoltaic batteries
11.5 Radiation damage in betavoltaic batteries
11.6 Radioisotopes for betavoltaic batteries
11.7 Betavoltaic batteries: results and analysis
11.8 Recent advances in betavoltaic batteries
11.9 Principles of betavoltaic battery design
11.10 Betavoltaic batteries for space applications
11.11 Conclusions
PART THREE Near earth and deep-space missions
12. Solar array designs for deep space science missions
Carolyn R. Mercer
12.1 Introduction
12.2 Modern missions using multiple wings or paddles
12.3 Missions using single solar panels
12.4 Missions using body-mounted solar arrays
12.5 Rideshare missions
12.6 Future trends
12.7 Conclusion
13. Lunar science based on Apollo solar cell measurements
S. Aranya, G. Gopkumar and T.E. Girish
13.1 Introduction
13.2 Solar cells used for the Apollo 14 dust detector experiment
13.3 Details of solar cell data used in this study
13.4 Variations of output of Apollo 14 solar cells within a lunation period
13.5 Annual variations of the solar cell output on the Moon
13.6 Solar proton events: Long-term degradation of Apollo 14 silicon solar cells
13.7 Discussion and analysis
13.8 Conclusions
14. Space photovoltaics for extreme high-temperature missions
Geoffrey A. Landis
14.1 Introduction
14.2 Solar cell operating temperature and efficiency
14.3 Temperature coefficient(s)
14.4 Approaches to solar arrays for near-Sun missions
14.5 Solar arrays with constant power at variable heliocentric distance
14.6 Thermal conversion for near-Sun missions
14.7 Earlier near-Sun missions
14.8 Parker Solar Probe
14.9 Photovoltaic power at Venus
14.10 Conclusions
15. Space photovoltaic concentrators for outer planet and near-Sun missions using ultralight Fresnel lenses
Mark J. O’Neill
15.1 Introduction and summary
15.2 A brief history of space PV concentrator technology
15.3 Description of the latest space fresnel lens photovoltaic concentrators
15.4 Recent lens developments
15.5 Recent multijunction cell developments
15.6 Recent graphene radiator developments
15.7 Performance metrics and cost savings
15.8 Conclusions
16. Technological relevance and photovoltaic production potential of high-quality silica deposits on Mars
T.E. Girish, Thara N. Sathyan and Varnana M. Kumar
16.1 Introduction
16.2 Spatial distribution and silica content of high-quality deposits on Mars
16.3 Expected performance of Si solar cells on Mars
16.4 Potential of silicon solar cell manufacturing on Mars
16.5 Conclusions
17. Space nuclear power: radioisotopes, technologies, and the future
Modeste Tchakoua Tchouaso, Tariq Rizvi Alam and Mark Antonio Prelas
17.1 Introduction
17.2 Radioisotope availability
17.3 Radioisotope power systems
17.4 Practical aspects of SNP systems
17.5 Conclusions: future of nuclear power technologies
- No. of pages: 534
- Language: English
- Edition: 1
- Published: October 26, 2022
- Imprint: Elsevier
- Paperback ISBN: 9780128233009
- eBook ISBN: 9780128233016
SB
Sheila G. Bailey
AH
Aloysius F. Hepp
Aloysius F. Hepp is Chief Technologist at Nanotech Innovations and an independent consultant based in Cleveland, Ohio. He earned a PhD in Inorganic Photochemistry in 1983 from MIT and retired in December 2016 from the Photovoltaic & Electrochemical Systems Branch of the NASA Glenn Research Center (Cleveland). He was a visiting fellow at Harvard University from 1992–3. He was awarded the NASA Exceptional Achievement medal in 1997. He has served as an adjunct faculty member at the University of Albany and Cleveland State University. Dr. Hepp has co-authored nearly 200 publications (including six patents) focused on processing of thin film and nanomaterials for I–III–VI solar cells, Li-ion batteries, integrated power devices and flight experiments, and precursors and spray pyrolysis deposition of sulfides and carbon nanotubes. He has co-edited twelve books on advanced materials processing, energy conversion and electronics, biomimicry, and aerospace technologies. He is Editor-in-Chief Emeritus of Materials Science in Semiconductor Processing (MSSP) and is currently the chair of the International Advisory Board of MSSP, as well as serving on the Editorial Advisory Boards of Mater. Sci. and Engin. B and Heliyon. He has recently been appointed as Series Editor for the Vacuum and Thin-Film Deposition Technologies series and the Aerospace Fundamentals, Applications, and Exploration series.
DF
Dale Ferguson
RR
Ryne P. Raffaelle
SD