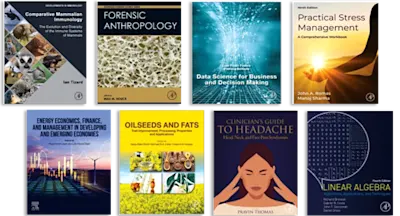
Optimizing Thermal, Chemical, and Environmental Systems
- 1st Edition - November 13, 2017
- Imprint: Elsevier
- Authors: Stanislaw Sieniutycz, Zbigniew Szwast
- Language: English
- Paperback ISBN:9 7 8 - 0 - 1 2 - 8 1 3 5 8 2 - 2
- eBook ISBN:9 7 8 - 0 - 1 2 - 8 1 3 5 8 3 - 9
Optimizing Thermal, Chemical and Environmental Systems treats the evaluation of power or energy limits for processes that arise in various thermal, chemical and environme… Read more
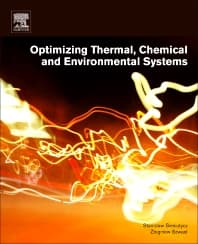
Purchase options
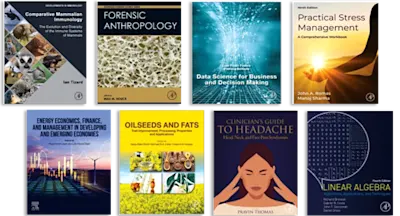
Institutional subscription on ScienceDirect
Request a sales quoteOptimizing Thermal, Chemical and Environmental Systems treats the evaluation of power or energy limits for processes that arise in various thermal, chemical and environmental engineering systems (heat and mass exchangers, power converters, recovery units, solar collectors, mixture separators, chemical reactors, catalyst regenerators, etc.). The book is an indispensable source for researchers and students, providing the necessary information on what has been achieved to date in the field of process optimization, new research problems, and what kind of further studies should be developed within quite specialized optimizations.
- Summarizes recent achievements of advanced optimization techniques
- Links exergy definitions in reversible systems with classical problems of extremum work
- Includes practical problems and illustrative examples to clarify applications
- Provides a unified description of classical and work-assisted heat and mass exchangers
- Written by a first-class expert in the field of advanced methods in thermodynamics
Scientists in academia and industry, chemical engineers, and students in (electro)chemistry, biotechnology, and ecology
1. Outline of Classical Optimization Methods 1.1 Applying Mathematical and Engineering Knowledge in Optimization 1.2 Unconstrained Problems for Function of Several Variables 1.3 Equality Constraints and Lagrange Multipliers 1.4 Methods of Mathematical Programming 1.5 Methods of Dynamic Optimization 1.6 Iterative Search Approaches 1.7 Some Stochastic Optimization Techniques
2. Finite Rate Optimization of Steady Thermal Units 2.1 Optimization Syntheses Toward Thermodynamic Limits 2.2 Maximizing Power Produced by a Finite Resource at Flow 2.3 Maximizing Cumulative Power 2.4 Discussion and Concluding Remarks
3. Neural Networks for Emission Prediction of Dust Pollutants 3.1 Introduction 3.2 Aims, Scope, and Assumptions 3.3 Experimental Data 3.4 Artificial Neural Networks 3.5 Emission Prediction With Multilayer Perceptron Method 3.6 Working Parameters of the Artificial Neural Network Model 3.7 Prediction Results 3.8 Emission Prediction by a Hybrid Model 3.9 Work Parameters of the Radial Base Function Network 3.10 Prediction Results 3.11 Summary and Conclusions
4. Neural NetworksdA Review of Applications 4.1 Introduction: General Issues 4.2 Training, Modeling, and Simulation 4.3 Performance Prediction, Optimization, and Related Issues 4.4 Hybrid and Fuzzy Systems
5. Uncontrolled FluideSolid Systems in Chemistry 5.1 Mass Penetration 5.2 Heterogeneous Process Regimes 5.3 GaseSolid Noncatalytic Reactions 5.4 Kinetic Analysis of Contact (Catalytic) Reactions (Szarawara et al., 1991, Chapter 5) 5.5 Kinetics of Surface Process 5.6 External Diffusion 5.7 Internal Diffusion 5.8 Catalyst Deactivation 5.9 Cascades of Tank Reactors 5.10 Reactor With the Product Recycle 5.11 Chemical Networks
6. Maximum Power in Homogeneous Chemical Systems 6.1 Introduction 175 6.2 Macro-Kinetics of Transport Phenomena and Chemical Reactions 6.3 Equations of Nonlinear Macro-Kinetics 6.4 Correspondence With Classical Chemical Kinetics 6.5 Inclusion of Nonlinear Transport Phenomena 6.6 Continuous Description of Chemical Kinetics and Transport 6.7 Principles of Power Production in Chemical Systems 6.8 Power Yield in Nonisothermal Chemical Engines 6.9 Nonisothermal Engines in Terms of Carnot Variables 6.10 Entropy Production in Steady Systems 6.11 Dynamical Dissipative Availabilities 6.12 Characteristics of Steady Isothermal Engines 6.13 Plausible Models of Dynamic Power Generators 6.14 Computational Algorithm for the Dynamical Process 6.15 Results of Computations 6.16 Some Additional Comments 6.17 Complex Systems With Internal Dissipation
7. Maximum Conversion in Processes With Chemical Reactions 7.1 Optimal Temperature Profile for a Single Reversible Chemical Reaction 7.2 Optimization of Consecutive Reactions in a Batch or Tubular Reactor 7.3 Parallel and Consecutive-Parallel Reactions in a Tubular or Batch Reactor
8. Reactors With Catalyst Decay and Regeneration 8.1 Mathematical Model for Kinetics of Reaction and Deactivation 8.2 Optimal Temperature Strategy for Single Catalytic Reaction in a Batch Reactor and Moving Bed Reactors 8.3 Tubular Reactor With Fixed Catalyst Bed and Optimal ReactioneRegeneration Cycle 8.4 Discussion 8.5 System of Consecutive-Parallel Reactions in Reactors With Catalyst Deactivation
9. Fuel Cells and Other Electrochemical Systems 9.1 Introduction 9.2 Electrochemical Engines 9.3 Entropy Production and Power Limits in Fuel Cells 9.4 Calculation of Operational Voltage 9.5 Thermodynamic Account of Current-Dependent and -Independent Imperfections 9.6 Evaluation of Mass Flows, Power Output, and Efficiency 9.7 Quality Characteristics and Feasibility Criteria 9.8 Some Experimental Results 9.9 Evaluating Power Limits in Thermo-Electro-Chemical Engines 9.10 Hybrid Systems 9.11 Unsteady States, Dynamic Units, and Control Problems 9.12 Biological Fuel Cells and Hydrogen Sources 9.13 Anode-Supported Solid Oxide Fuel Cell for Determination of Poisoning Limits
10. Optimizing Circulation Reactor With Deactivating Catalyst 10.1 Introducing the Problem of Optimal Temperatures in Circulation Reactors 10.2 Formulation of the Optimization Problem 10.3 Shapes of Optimal Temperature Profiles 10.4 Results of Numerical Calculations 10.5 Summarizing Remarks
11. Optimizing Reactore - Regenerator System With Catalytic Parallel-Consecutive Reactions 11.1 Introduction 11.2 Mathematical Model of Catalyst Deactivation 11.3 Mathematical Model of Chemical Reactions 11.4 Process Profit Flux 11.5 Optimization Problem and Computational Algorithm 11.6 Some Results 11.7 Conclusions
12. Maximum Principle and Other Criteria of Dynamic OptimizationdAn Unconventional Approach 12.1 Introducing the Standard Form of the Continuous Optimization Problem 12.2 Dynamic Programming Investigation of Optimal Quality Function 12.3 Continuous Maximum Principle 12.4 Solving Methods for Maximum Principle Equations 12.5 Discrete Versions of Maximum Principle 12.6 Classification and Comparison of Various Computational Methods for Optimization of Functionals
- Edition: 1
- Published: November 13, 2017
- No. of pages (Paperback): 452
- No. of pages (eBook): 452
- Imprint: Elsevier
- Language: English
- Paperback ISBN: 9780128135822
- eBook ISBN: 9780128135839
SS
Stanislaw Sieniutycz
ZS