Limited Offer
Save 50% on book bundles
Immediately download your ebook while waiting for your print delivery. No promo code needed.
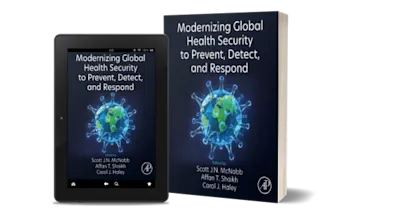
The use of animal models is a key aspect of scientific research in numerous fields of medicine. Movement Disorders, Second Edition vigorously examines the important contribut… Read more
Limited Offer
The use of animal models is a key aspect of scientific research in numerous fields of medicine. Movement Disorders, Second Edition vigorously examines the important contributions and application of animal models to the understanding of human movement disorders, and serves as an essential resource for basic neuroscientists engaged in movement disorders research. Academic clinicians, translational researchers and basic scientists are brought together to connect experimental findings made in different animal models to the clinical features, pathophysiology and treatment of human movement disorders.
The book is divided into sections on Parkinson's disease, Huntington's disease, dystonia, tremor, paroxysmal movement disorders, ataxia, myoclonus, restless legs syndrome, drug-induced movement disorders, multiple system atrophy, progressive supranuclear palsy/corticobasal degeneration, and spasticity. This book serves as an essential resource for both clinicians interested in the science being generated with animal models and basic scientists studying the pathogenesis of particular movement disorders.
Section I. Scientific Foundations
Section II. Parkinson Disease
Section III. Dystonia
Section IV. Huntington Disease
Section V. Tremor
Section VI. Myoclonus
Section VII. Tics
Section VIII. Paroxysmal Movement Disorders
Section IX. Tauopathies
Section X. Other Parkinsonian Syndromes: NBIA, MSA, PD + Spasticity, PD + Dystonia
Section XI. Ataxias
Section XII. Hereditary Spastic Paraplegia
Section XIII. Restless Legs Syndrome
ML