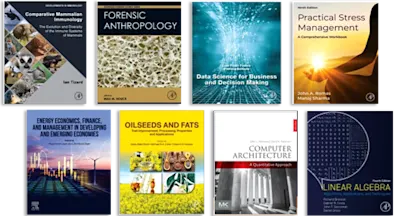
Microalgal Biofuels
Sustainable Production, Conversion, and Life Cycle Assessment
- 1st Edition - January 29, 2025
- Imprint: Woodhead Publishing
- Editors: Krishna Kumar Jaiswal, Bhaskar Singh, Amit K. Jaiswal
- Language: English
- Paperback ISBN:9 7 8 - 0 - 4 4 3 - 2 4 1 1 0 - 9
- eBook ISBN:9 7 8 - 0 - 4 4 3 - 2 4 1 1 1 - 6
Microalgal Biofuels: Sustainable Production and Conversion is a comprehensive guide to the latest advancements in microalgal biofuels. The book provides systematic coverage… Read more
Purchase options
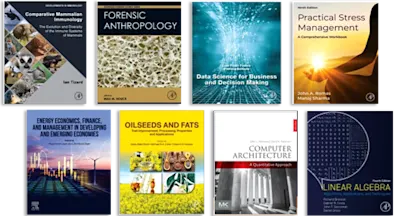
Microalgal Biofuels: Sustainable Production and Conversion is a comprehensive guide to the latest advancements in microalgal biofuels. The book provides systematic coverage of the processes of biofuel production, from microalgae biomass resources to biomass conversion processes and catalytic materials. It delves into the critical topic of sustainability, addressing LCA approaches to evaluate the environmental impacts of microalgal-based biofuels. It provides practical information and guidance on the latest strategies, opportunities, and challenges in the transition to sustainable bioenergy. This is an invaluable reference for students, researchers, and industrial practitioners working on biofuels, biotechnology, bioprocess engineering, and biomass conversion.
Divided into four sections, the first section introduces the principles of microalgal biology and cultivation, including an overview of the different types of microalgae, their growth requirements, and the cultivation systems used for large-scale production. The second section explains the conversion of microalgal biomass into biofuels, including biodiesel, bioethanol, biogas, and hydrogen. Each chapter in this section covers a different biofuel pathway, highlighting the technological advancements, challenges, and opportunities for scaling up production. The third section of the book explores the sustainability aspects of microalgal biofuel production, including the use of waste streams and the integration of biofuel production with other industries. This section also covers the LCA approaches used to evaluate the environmental impacts of microalgal biofuels and the strategies for enhancing their sustainability. The fourth and final section of the book examines the commercialization and prospects of microalgal biofuels. This section covers the market potential of microalgal biofuels, the regulatory landscape, and the challenges and opportunities for the industry.
- Presents the latest advancements in microalgal-based biofuel production and sustainability
- Offers comprehensive coverage of microalgal biomass resources, conversion processes, and catalytic materials
- Provides sustainability and environmental impacts using lifecycle assessment (LCA) approaches
- Includes a detailed analysis of the commercialization and prospects of microalgal biofuels
Students, researchers, and industry engineers involved in bioenergy and biofuel. It will include those with an interest in bioenergy, microalgal biofuels, algal biotechnology, bioprocess engineering, and biomass conversion, Environmental and sustainability professionals interested in the LCA-focused chapters
1. Introduction to biofuels and microalgal biofuels
2. Strategies for bioenergy and biofuels from algae biomass
3. Algal farming approaches: Raceway ponds and photobioreactor for biomass generation
4. Non-cellulosic and lignocellulosic biomass for biofuels
5. Biochemical molecules of microalgae and their functional energy applications
6. Biorefinery approach for biofuels to obtain high-value chemicals from microalgae
7. Opportunities and challenges in the transition of microalgae biomass to biofuels
Section – II: Biomass Conversion Processes
8. Bioprocess engineering and Bioenergy conversion systems
9. Biochemical conversions of microalgae biomass for biofuel production
10. Thermochemical conversion of microalgae biomass for biofuel production
11. Microalgal bio-electrochemical conversions of biohydrogen fuels
12. Waste-to-biofuel conversion technologies
13. High-value chemicals from biomass conversion processes
Section – III: Catalytic Materials for Biomass to Biofuels Conversion
14. Catalytic materials for biomass to biofuels conversion-
15. Enzymatic deconstruction of complex biomass for organic molecules
16. Biocatalysts for hydrolysis and liquefaction of lignocellulosic biomass
17. Catalytic materials for thermochemical conversions process for biofuels
18. Novel approaches in cost-reduction and scale-up of biofuel production
Section IV: Sustainability in Algae Biofuels Production
19. Environmental impacts of algae biofuels production
20. Applications of microalgal biofuels
21. Social and economic impacts of algae biofuels production
22. Climate change mitigation potential of algae biofuels
23. Sustainability certification and standards for algae biofuels production
24. Life cycle assessment of algae biofuels or LCA approaches in microalgal based biofuels towards sustainability and carbon neutrality
25. Case studies on microalgal biofuels status and exploration
- Edition: 1
- Published: January 29, 2025
- Imprint: Woodhead Publishing
- Language: English
KJ
Krishna Kumar Jaiswal
Dr. Krishna Kumar Jaiswal obtained his Ph.D. in Green Energy Technology and postdoctoral experiences with a broad area of research in bioenergy and biofuels. He is working as an Assistant Professor at Pondicherry University, India. Dr. Krishna's research and teaching focus on bioprocess engineering, nanotechnology, and biofuels technologies in the Department of Green Energy Technology. He is actively researching and developing new technologies for bioprocessing and biofuels. He is also a subject expert for the National Testing Agency (Govt. of India), India. He has published over 65+ research papers in reputed journals and is an active life member of several renowned scientific societies, an editorial board member, and a reviewer of several reputed scientific journals. He also serves as a member of Boards of Studies, various Doctoral Committees, and other Administrative Positions for different Universities.
BS
Bhaskar Singh
AJ
Amit K. Jaiswal
Dr. Amit K. Jaiswal is an esteemed academic and researcher currently serving as a Lecturer at the School of Food Science and Environmental Health, Technological University Dublin (TU Dublin)—City Campus, Ireland. Recognised globally for his scholarly contributions, Dr Jaiswal has been recognised among the top 1% of the world’s most cited academics in 2023 and 2024 by Clarivate Analytics, a distinction given to researchers who have demonstrated exceptional influence in their fields over the past decade. In addition, Stanford University has listed him among the top 2% of scientists worldwide for four consecutive years (2021–24). Dr Jaiswal’s research focuses on converting lignocellulosic biomass and algae (micro- and macroalgae) into biofuels, biomaterials, and biochemicals through innovative process development, techno-economic analysis, and life cycle assessment. He brings extensive expertise in bio-based materials, such as lignin and microcellulose/nanocellulose, and their applications in sustainable food packaging, water purification, and adhesives. His proficiency in green extraction techniques, including deep eutectic solvents (DES) and ultrasound-assisted processes, enables the valorisation of agri-food biomass into high-value products. With more than 125 peer-reviewed publications, 50 book chapters, and five edited books, Dr. Jaiswal’s contributions to scientific literature have significantly impacted food science and biotechnology. His work has received over 10,000 citations, with an h-index exceeding 50. He also serves on the editorial boards of key international journals, including Food Quality and Safety (Oxford University Press), Foods, Biomass (MDPI) and JSFA Reports (Wiley).