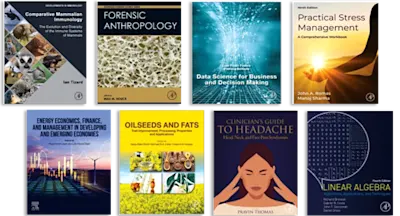
Metal Cutting
Theory, Selection, and Design
- 5th Edition - April 18, 2025
- Imprint: Butterworth-Heinemann
- Authors: Edward M. Trent, Paul K. Wright, Peter A. Dearnley
- Language: English
- Paperback ISBN:9 7 8 - 0 - 3 2 3 - 9 9 1 5 5 - 1
- eBook ISBN:9 7 8 - 0 - 3 2 3 - 9 9 1 5 7 - 5
Metal Cutting, Fifth Edition builds upon the classic work that has for decades been the go-to reference for individuals working in the area of metal cutting. This revised editio… Read more
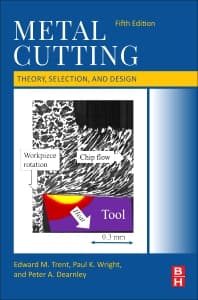
Purchase options
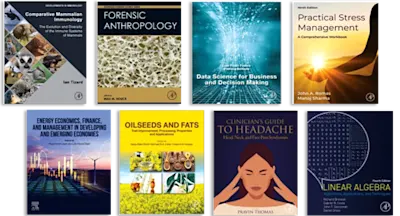
Institutional subscription on ScienceDirect
Request a sales quote- Dispels misconceptions concerning the cutting tool–workpiece interface interaction during turning, milling, and drilling operations as well as those concerning the structure and properties of cutting tool materials
- Clarifies the reality of cutting tool wear mechanisms and shows how their complexity depends on the rates of metal removal and the properties of the workpiece being machined
- Outlines best practices for the determination and evaluation of cutting tool wear and shows how to determine and investigate tool contact stresses, temperatures, and chip (swarf) formation in metal cutting
- Metal Cutting
- Cover image
- Title page
- Table of Contents
- Copyright
- Dedication
- Preface
- Part I: The Basics
- Chapter 1 Introduction
- Abstract
- Keywords:
- 1.1 The Metal Cutting (or Machining) Process
- 1.2 A Short History of Machining
- 1.3 Machining and the Global Economy
- 1.4 Summary and Conclusions
- 1.4.1 Economics
- 1.4.2 Sociology
- 1.4.3 Technology
- References
- Chapter 2 Metal Cutting Operations and Terminology
- Abstract
- Keywords:
- 2.1 Summary Points
- 2.2 Introduction
- 2.3 Turning or Turn-Cutting
- 2.4 Boring
- 2.5 Drilling
- 2.6 Facing
- 2.7 Forming and Parting Off
- 2.8 Milling or Mill-Cutting
- 2.9 Shaping and Planing
- 2.10 Broaching
- 2.11 Final Perspective
- References
- Bibliography (Also See Chapter 16)
- Chapter 3 The Essential Features of Metal Cutting
- Abstract
- Keywords
- 3.1 Summary Points
- 3.2 Introduction
- 3.3 The Chip
- 3.4 Techniques for Studying Chip Formation
- 3.5 Chip Shape
- 3.6 Chip Formation
- 3.7 The Chip/Tool Interface
- 3.8 Chip Flow Under Conditions of Seizure
- 3.9 The Built-Up Edge
- 3.10 Machined Surfaces
- 3.11 Conclusions
- References
- Chapter 4 Forces and Stresses in Metal Cutting
- Abstract
- Keywords
- 4.1 Summary Points
- 4.2 Introduction
- 4.3 Forces and Stresses on the Primary Shear Plane
- 4.4 Forces in the Flow Zone
- 4.5 The Primary Shear Plane and Minimum Energy Theory
- 4.5.1 Merchant’s shear “plane” analysis
- 4.5.2 Oxley’s shear “zone” analysis
- 4.5.3 Rowe and Spick’s approximate analysis
- 4.6 Forces in Cutting Metals and Alloys
- 4.7 Stresses in the Tool
- 4.8 Stress Distribution
- 4.8.1 Introduction
- 4.8.2 Experiments with split tools – Method 1
- 4.8.3 Experiments with tools of different strengths – Method 2
- 4.8.4 Experiments with photo-elastic polymers – Method 3
- 4.8.5 Experiments with photo-elastic sapphire – Method 4
- 4.8.6 Normal stress distribution: Typical results
- 4.8.7 Shear stress distribution: Typical results
- 4.8.8 General summary of photo-elasticity work and stress analyses
- 4.8.9 A special note on practical machining with ceramics and diamond tools
- 4.9 Conclusions
- References
- Chapter 5 Heat in Metal Cutting
- Abstract
- Keywords:
- 5.1 Summary Points
- 5.2 Introduction
- 5.3 Heat in the Primary Shear Zone
- 5.4 Heat at the Tool/Work Interface
- 5.4.1 Interface temperatures with a BUE
- 5.4.2 Interface temperatures with a flow zone
- 5.4.3 Calculation of interface temperatures with a flow zone
- 5.5 Heat Flow at the Tool Clearance Face
- 5.6 Heat in Areas of Sliding
- 5.7 Methods of Tool Temperature Measurement
- 5.7.1 Tool/work thermocouple
- 5.7.2 Inserted thermocouples
- 5.7.3 Radiation methods
- 5.7.4 Changes in hardness and microstructure in steel tools
- 5.8 Measured Temperature Distribution in Tools
- 5.9 Relationship of Tool Temperature to Speed
- 5.10 Relationship of Tool Temperature to Tool Design
- 5.11 Conclusions
- References
- Part II: The Tool Materials
- Chapter 6 High-Speed Steels
- Abstract
- Keywords:
- 6.1 Summary Points
- 6.2 Background and History
- 6.3 High-Carbon Steel Tools
- 6.4 High-Speed Steels
- 6.5 Structure and Composition
- 6.5.1 Tungsten and molybdenum
- 6.5.2 Carbon
- 6.5.3 Chromium
- 6.5.4 Vanadium
- 6.5.5 Cobalt
- 6.6 Properties of High-Speed Steels
- 6.7 Wear Mechanisms of Uncoated High-Speed Steel Tools
- 6.7.1 How to examine used cutting tools
- 6.7.2 Superficial (discrete) plastic deformation by shear at high temperature
- 6.7.3 Plastic deformation of the cutting edge under compressive stress
- 6.7.4 Dissolution/diffusion wear
- 6.7.5 Attrition wear (plucking or grain pull-out)
- 6.7.6 Abrasive wear
- 6.7.7 Wear under sliding conditions
- 6.7.8 Summary
- 6.8 Tool-Life Testing
- 6.9 Conditions of Use
- 6.10 Coated High-Speed Steel Tools
- 6.11 Other Developments
- 6.12 Concerning Powder Metallurgy (PM) High-Speed Steel
- 6.13 Conclusions
- References
- Chapter 7 Uncoated Cemented Carbides
- Abstract
- Keywords:
- 7.1 Summary Points and Background
- 7.2 Structures and Properties of Cemented Carbides
- 7.3 Straight-Grade Tungsten Carbide-Cobalt Alloys (WC-Co)
- 7.3.1 Background
- 7.3.2 Stress-strain behavior
- 7.3.3 Fracture strength
- 7.3.4 Fracture toughness
- 7.3.5 Hardness (H), yield strength (σy), and 0.2% proof strength (σ0.2%)
- 7.3.6 Young’s modulus and the rules of mixtures
- 7.3.7 Density
- 7.3.8 Further comments on the hardness (H) of cemented carbides
- 7.4 Steel Cutting Grade WC-γC-Co Alloys
- 7.4.1 General perspective
- 7.4.2 More concerning microstructure, composition, and properties of WC-γC-Co alloys compared to WC-Co alloys
- 7.5 Elevated Temperature and Other Thermal Properties
- 7.5.1 High-temperature mechanical properties of WC-Co and WC-γC-Co alloys
- 7.5.2 Other thermal properties of WC-Co and WC-γC-Co alloys
- 7.6 Metal Removal Rates and Machining Charts
- 7.7 Wear Mechanisms of Uncoated Cemented Carbides
- 7.7.1 Superficial (discrete) plastic deformation by shear at high temperature
- 7.7.2 Plastic deformation of the cutting edge under compressive stress
- 7.7.3 Diffusion wear or dissolution/diffusion wear (DDW)
- 7.7.4 Attrition wear (plucking or grain pull-out)
- 7.7.5 Abrasive wear
- 7.7.6 Fracture
- 7.7.7 Thermal fatigue and thermal stress
- 7.7.8 Notch wear (NW) or grooving wear (under sliding conditions)
- 7.7.9 Summary
- 7.8 Titanium Cermets
- 7.9 Laminated Carbide Tools
- 7.10 Techniques of Using Cemented Carbides for Cutting
- 7.10.1 Early methods using brazed-on carbide tips
- 7.10.2 The transition to throw-away tool tips or indexable inserts
- 7.11 Overall Summary
- References
- Chapter 8 Coated Cutting Tools
- Abstract
- Keywords
- 8.1 Summary Points and Background
- 8.2 Coating Technologies
- 8.2.1 Chemical vapor deposition (CVD)
- 8.2.2 Plasma-assisted CVD (PACVD), also termed plasma-enhanced CVD (PECVD)
- 8.2.3 Physical vapor deposition (PVD)
- 8.3 Wear Mechanisms and Wear Rates of Coated Cemented Carbides
- 8.3.1 Background
- 8.3.2 Rake face and flank face wear rates
- 8.3.3 Rake face and flank face wear mechanisms
- 8.3.4 Coating breakthrough and coated tool wear patterns
- 8.3.5 Textured CVD-Al2O3 coated cemented carbides
- 8.3.6 Adhesive fracture wear (AFW) on the rake and flank faces
- 8.3.7 Notch wear of coated cemented carbides
- 8.3.8 Notch wear of coated and uncoated cermets
- 8.3.9 Thermal stress
- 8.4 Summary
- 8.5 Terminology
- References
- Chapter 9 Alumina (Al2O3)-Based Ceramics and Sialons
- Abstract
- Keywords:
- 9.1 Summary Points
- 9.2 Background
- 9.3 Alumina (Al2O3)-Based Ceramics
- 9.3.1 Alumina ceramics
- 9.3.2 Zirconia-toughened alumina (ZTA) composites
- 9.3.3 Alumina-titanium carbide (Al2O3-TiC) and related mixed ceramic composites
- 9.3.4 Alumina-silicon carbide whisker (Al2O3-SiCw) reinforced composites
- 9.3.5 Cutting edge geometry
- 9.4 Sialon Ceramics
- 9.4.1 Microstructure and properties of sialons
- 9.4.2 Further aspects of sialon tool wear
- 9.4.3 Sialons with an additional phase or surface modification
- 9.5 Summary
- References
- Chapter 10 Ultrahard Tool Materials
- Abstract
- Keywords:
- 10.1 Summary Points and Background
- 10.2 Cubic Boron Nitride (CBN)
- 10.2.1 General properties of CBN
- 10.2.2 Machining performance of CBN
- 10.3 Diamond, Synthetic Diamond, and Diamond-Coated Cutting Tools
- 10.3.1 General properties of diamond
- 10.3.2 Synthetic diamond and polycrystalline diamond (PCD) composites
- 10.3.3 Machining performance of synthetic PCD diamond tools
- 10.3.4 CVD polycrystalline diamond- and DLC-coated tools
- References
- Part III: Applying the Tools
- Chapter 11 Machinability of Steels and Cast Irons
- Abstract
- Keywords
- 11.1 Summary Points
- 11.2 Defining Machinability (MTY)
- 11.2.1 Temperature effects
- 11.2.2 Structure of this chapter and Chapter 12
- 11.3 Commercially Pure Iron
- 11.4 Steels: Alloy Steels and Heat Treatments
- 11.4.1 Influence of alloying elements on the forces and stresses on the tool
- 11.4.2 Relative MTY of steel
- 11.4.3 Combined influence of alloying, heat treatment, stress, and temperature
- 11.4.4 BUE effects
- 11.5 Free Cutting Steels
- 11.5.1 Economics
- 11.5.2 Resulfurized free cutting steels
- 11.5.3 The role of lead additions
- 11.5.4 Other factors that influence the machinability of some steels
- 11.6 Hard Steels and Cast Irons (Room Temperature HV > 450 kg/mm2)
- 11.7 Austenitic Stainless Steels
- 11.7.1 Standard austenitic and duplex stainless steels
- 11.7.2 Free cutting stainless steels
- 11.8 Cast Iron
- 11.8.1 Gray cast iron microstructures and chip formation
- 11.8.2 Aspects of machining gray cast irons
- 11.8.3 Alloy and chilled cast irons
- 11.9 Conclusions
- References
- Chapter 12 Machinability of Other Metals
- Abstract
- Keywords:
- 12.1 Summary Points
- 12.2 Magnesium
- 12.3 Aluminum and Aluminum Alloys
- 12.3.1 Introduction
- 12.3.2 Machinability of aluminum-silicon (Al-Si) alloys
- 12.3.3 Aluminum alloys versus commercially pure aluminum
- 12.3.4 Some industrial/practical constraints
- 12.3.5 Free machining aluminum alloys
- 12.3.6 Summary on aluminum alloys
- 12.4 Copper, Brass, and Other Copper Alloys
- 12.4.1 Introduction
- 12.4.2 Machinability of conventional brasses
- 12.4.3 Machinability of free machining brass
- 12.4.4 Environmental concerns
- 12.4.5 Aluminum bronze
- 12.4.6 Cupro-nickel alloys
- 12.4.7 Summary on copper, brass, and copper-based alloys
- 12.5 Nickel and Its Alloys
- 12.5.1 Commercially pure nickel
- 12.5.2 Nickel alloys
- 12.6 Titanium and Titanium Alloys
- 12.6.1 Background summary
- 12.6.2 Chip formation
- 12.6.3 Commercial purity titanium (CP-Ti)
- 12.6.4 Titanium alloys
- 12.6.5 Alternative tool materials for machining titanium alloys
- 12.7 Zirconium
- 12.8 Conclusions
- References
- Chapter 13 Coolants and Lubricants
- Abstract
- Keywords
- 13.1 Summary Points
- 13.2 Introduction
- 13.2.1 Cooling effects of water-based cutting fluids
- 13.2.2 Lubricating effects of neat cutting oil fluids
- 13.2.3 Selection for different processes and work material types
- 13.2.4 Dry versus water-based versus oil-based fluids
- 13.3 Coolants
- 13.3.1 Importance of fluid jet and nozzle location
- 13.3.2 Machining steel with coolants
- 13.3.3 Machining commercially pure nickel with coolants
- 13.3.4 Practical considerations with coolants
- 13.3.5 Potential use of high-pressure jets and carbon dioxide
- 13.3.6 A special experiment with liquid nitrogen
- 13.4 Lubricants
- 13.4.1 Conditions of seizure impede the action of external lubricants
- 13.4.2 Lubrication at the periphery of contact or during intermittent cutting
- 13.4.3 Experiments in controlled atmospheres
- 13.4.4 Importance of “natural air” as a lubricant
- 13.4.5 Importance of nitrogen in protecting tool surfaces
- 13.4.6 Summarizing the role of air in cutting and implications for dry cutting
- 13.4.7 Extreme pressure additives in lubricants
- 13.4.8 Surface finish
- 13.4.9 Influence of cutting speed and tool material type
- 13.5 Conclusions on Coolants and Lubricants
- 13.5.1 General observations
- 13.5.2 Trends toward dry cutting
- 13.5.3 Recommendations
- References
- Chapter 14 High-Speed Machining
- Abstract
- Keywords:
- 14.1 Summary Points
- 14.2 Introduction to High-Speed Machining
- 14.3 Economics of High-Speed Machining
- 14.4 Brief Historical Perspective
- 14.5 Influence of Increasing Speed on Chip Type
- 14.5.1 The main chip types
- 14.5.2 Transition from type C to type D chip forms
- 14.6 Austenitic Stainless Steel
- 14.6.1 Overview
- 14.6.2 Photomicrographs of quick stops
- 14.6.3 Proposed mechanism for type D segmented chips in stainless steel
- 14.6.4 Summary of the above effects
- 14.7 Hardened and Tempered AISI 4340
- 14.7.1 Overview
- 14.7.2 Conditions in the primary zone when machining AISI 4340 steel
- 14.8 Aerospace Aluminum and Titanium
- 14.8.1 Overview
- 14.8.2 Cutting Ti-6A1-4V at high speeds
- 14.8.3 Cutting aluminum alloys at high speeds
- 14.9 Discussion and Recommendations
- 14.9.1 Segmented chip forms in high-speed machining
- 14.9.2 Tool forces
- 14.9.3 Tool wear
- 14.10 Conclusions
- References
- Part IV: Student Tasks and Bibliography
- Chapter 15 Student Exercises
- Abstract
- Keywords
- 15.1 Part A – Questions Are Based on Section 7.2 of Chapter 7
- 15.2 Part B – Questions Based on Other Chapters
- 15.3 Part C – A Tutorial on Machining Economics
- Chapter 16 Bibliography and Selected Web-Sites
- 16.1 Introduction
- 16.2 Texts and on Metal Cutting and Tool Materials
- 16.3 General Texts That Include Metal Cutting
- 16.4 Selected Texts on Rapid Prototyping
- 16.5 Selected Texts on Forming
- 16.6 Suggested Background Reading
- 16.7 Recommended Journals
- 16.8 Web-Site Resources and Technical Bulletins of Tool Material Suppliers
- Index
- Edition: 5
- Published: April 18, 2025
- No. of pages (Paperback): 632
- No. of pages (eBook): 400
- Imprint: Butterworth-Heinemann
- Language: English
- Paperback ISBN: 9780323991551
- eBook ISBN: 9780323991575
ET
Edward M. Trent
PW
Paul K. Wright
Paul Kenneth Wright (PhD) is prominent in the field and was formerly a professor of mechanical engineering and co-chairman of the Management of Technology Program at the University of California/Berkeley School of Engineering. He has written numerous articles for journals, conferences, and symposiums, as well as coauthored "Manufacturing Intelligence," with D.A. Bourne. In addition, he has served as a consultant and has been involved in many professional organizations.
PD
Peter A. Dearnley
Peter Albany Dearnley (PhD) formerly: Visiting Professor University of Southampton, UK, Senior Lecturer University of Leeds, UK.; Senior Lecturer University of Auckland, NZ., Senior Research Associate University of Cambridge, Research Fellow University of Birmingham, Research Technologist Sandvik Hard Materials Ltd, UK. Awarded (with Mr A N Grearson of Sandvik Hard Materials) the 1987 "Pfeil Medal" by the Institute of Materials, in recognition of their major contribution to understanding cutting tool wear mechanisms when machining titanium alloys.