Medical Modeling
The Application of Advanced Design and Additive Manufacturing Techniques in Medicine
- 3rd Edition - June 8, 2024
- Authors: Richard Bibb, Dominic Eggbeer, Abby Paterson, Mazher Iqbal Mohammed
- Language: English
- Paperback ISBN:9 7 8 - 0 - 3 2 3 - 9 5 7 3 3 - 5
- eBook ISBN:9 7 8 - 0 - 3 2 3 - 9 5 7 3 4 - 2
Medical Modelling: The Application of Advanced Design and Rapid Prototyping Techniques in Medicine, Third Edition provides readers with a thorough update of the core conten… Read more
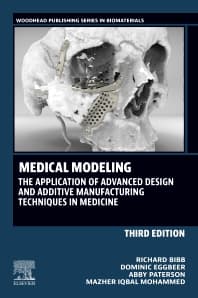
Purchase options
Institutional subscription on ScienceDirect
Request a sales quoteFinally, the book explores the future potential of medical modeling, such as in simulations for training, the development of new medical devices, and more.
- Covers the essential stages and methods of creating virtual and physical anatomical models from medical scan data
- Presents an overview of the main AM processes, including advantages and limitations
- Provides worked examples and case studies with detailed descriptions of the applications of 3D scanning, CAD, and AM to a wide variety of anatomical, surgical, prosthetic, orthotic, and associated applications
Researchers and postgraduate students in the fields of materials science, biomedical engineering, medical imaging and additive manufacturing, Clinical scientists and R&D groups interested in additive manufacturing and imaging techniques for medical modelling
- Cover image
- Title page
- Table of Contents
- Copyright
- About the authors
- Preface
- Acknowledgements
- Chapter 1. Introduction
- 1.1. Background
- 1.2. The human form
- 1.3. Basic anatomic terminology
- 1.4. Technical terminology
- Chapter 2. Medical imaging
- 2.1. Introduction to medical imaging
- 2.2. Computed tomography
- 2.3. Cone beam computed tomography
- 2.4. Magnetic resonance
- 2.5. Noncontact surface scanning
- 2.6. Medical scan data
- 2.7. Point cloud data
- 2.8. Media
- 2.9. Summary
- Chapter 3. Working with medical scan data
- 3.1. Image segmentation
- 3.2. Using CT data: Worked examples
- 3.3. Point cloud data operations
- 3.4. Two-dimensional formats
- 3.5. Pseudo 3D formats
- 3.6. True 3D formats
- 3.7. File management and exchange
- 3.8. Summary
- Chapter 4. Physical reproduction
- 4.1. Introduction to additive manufacturing
- 4.2. Vat polymerization
- 4.3. Material extrusion
- 4.4. Powder bed fusion
- 4.5. Material jetting
- 4.6. Computer numerical controlled machining
- 4.7. Cleaning and sterilizing medical models
- 4.8. Summary
- Chapter 5. Case studies
- 5.1. Introduction
- Part 5.1. Implementation
- Chapter 5.1. Implementation case study 1: Computed tomography guidelines for medical modeling using additive manufacturing techniques
- 5.1.1. Introduction
- 5.1.2. CT guidelines for medical modeling
- 5.1.3. Conclusion
- Chapter 5.2. Implementation case study 2: The evolving development of a collaborative service: Organizational, technical, and regulatory considerations
- 5.2.1. Introduction
- 5.2.2. The early years of collaboration—Establishing a joint medical modeling service
- 5.2.3. Service evolution toward greater in-hospital capability
- 5.2.4. Bringing greater design expertise and guide production capability in-hospital
- 5.2.5. Evolving regulatory considerations
- Chapter 5.3. Implementation case study 3: Medical additive manufacturing technologies: State of the art and current limitations for application in oral and maxillofacial surgery
- 5.3.1. Introduction
- 5.3.2. 3D image acquisition and processing for MRP
- 5.3.3. Rapid prototyping technologies
- 5.3.4. Medical rapid prototyped model artifacts
- 5.3.5. Conclusion
- Part 5.2. Surgical applications
- Chapter 5.4. Surgical application case study 1—Planning osseointegrated implants using computer-aided design and additive manufacturing
- 5.4.1. Introduction
- 5.4.2. The proposed approach
- 5.4.3. Scanning problems
- 5.4.4. Software problems
- 5.4.5. An illustrative case study
- 5.4.6. Results
- 5.4.7. Benefits and future development
- Chapter 5.5. Surgical applications case study 2—Rapid manufacture of custom fitting surgical guides
- 5.5.1. Introduction
- 5.5.2. Methods
- 5.5.3. Case study
- 5.5.4. Results
- 5.5.5. Discussion
- 5.5.6. Conclusions
- Chapter 5.6. Surgical application case study 3—The use of a reconstructed 3D solid model from CT to aid the surgical management of a total knee arthroplasty
- 5.6.1. Introduction
- 5.6.2. Materials and methods
- 5.6.3. Postoperative management and follow up
- 5.6.4. Discussion
- Chapter 5.7. Surgical application case study 4—The custom-made titanium orbital floor prosthesis in reconstruction for orbital floor fractures
- 5.7.1. Introduction
- 5.7.2. Technique
- 5.7.3. Case report
- 5.7.4. Conclusion
- Chapter 5.8. Surgical application case study 5—The use of 3D technology in the multidisciplinary management of facial disproportion
- 5.8.1. Introduction
- 5.8.2. Materials and method
- 5.8.3. Results
- 5.8.4. Discussion
- Chapter 5.9. Surgical applications case study 6—An appropriate approach to computer-aided design and manufacture of reconstructive implants
- 5.9.1. Introduction
- 5.9.2. Case 1—Orbital rim augmentation implant
- 5.9.3. Case 2—Orbital floor implant incorporating placement guide
- 5.9.4. Case study 3—Multipart reconstruction
- 5.9.5. Case 4—Posttraumatic zygomatic osteotomy and orbital floor reconstruction
- Chapter 5.10. Surgical application case study 7—Computer-aided planning and additive manufacture for complex, mid-face osteotomies
- 5.10.1. Introduction
- 5.10.2. Methods
- 5.10.3. Results
- 5.10.4. Discussion
- 5.10.5. Conclusions
- Chapter 5.11. Surgical applications case study 8—Virtual surgical planning and development of a patient-specific resection guide for treatment of distal radius osteosarcoma
- 5.11.1. Introduction
- 5.11.2. Methods
- 5.11.3. Results
- 5.11.4. Conclusion
- Part 5.3. Prosthetic applications
- Chapter 5.12. Prosthetic rehabilitation applications case study 1—An investigation of three-dimensional scanning of human body surfaces and its use in the design and manufacture of prostheses
- 5.12.1. Introduction
- 5.12.2. Methods
- 5.12.3. Results
- Chapter 5.13. Prosthetic rehabilitation applications case study 2—Producing burns therapy conformers using noncontact scanning and additive manufacturing
- 5.13.1. Introduction
- 5.13.2. Methods
- 5.13.3. Results
- 5.13.4. Discussion
- 5.13.5. Conclusions
- Chapter 5.14. Prosthetic rehabilitation applications case study 3—An appropriate approach to computer-aided design and manufacture of cranioplasty plates
- 5.14.1. Introduction
- 5.14.2. First case
- 5.14.3. Second case
- 5.14.4. Third case—Press tool design
- 5.14.5. Fourth case—Implant design for metal AM fabrication
- 5.14.6. Fifth case—Implant design for PEEK AM fabrication
- 5.14.7. Future development and benefits
- Chapter 5.15. Prosthetic rehabilitation applications case study 4—Evaluation of advanced technologies in the design and manufacture of an implant-retained facial prosthesis
- 5.15.1. Introduction
- 5.15.2. Existing facial prosthetics technique
- 5.15.3. Review of advanced technologies in facial prosthetics
- 5.15.4. Case 1
- 5.15.5. Case 2
- 5.15.6. Results
- 5.15.7. Discussion
- 5.15.8. Conclusions
- Chapter 5.16. Prosthetic rehabilitation applications case study 5—Additive manufacturing technologies in soft tissue facial prosthetics: Current state of the art
- 5.16.1. Introduction
- 5.16.2. Methodology
- 5.16.3. Summary of case studies
- 5.16.4. Discussion
- 5.16.5. AM specification
- 5.16.6. Conclusions
- Chapter 5.17. Prosthetic rehabilitation applications case study 6—Evaluation of direct and indirect additive manufacture of maxillofacial prostheses using additive manufacturing
- 5.17.1. Introduction
- 5.17.2. Methods
- 5.17.3. Results
- 5.17.4. Discussion
- 5.17.5. Conclusions
- Acknowledgements
- Chapter 5.18. Prosthetic rehabilitation applications case study 7—Computer-aided methods in bespoke breast prosthesis design and fabrication
- 5.18.1. Introduction
- 5.18.2. Methods
- 5.18.3. Discussion
- 5.18.4. Conclusions
- Chapter 5.19. Prosthetic rehabilitation applications case study 8—Immediate nasal prosthesis following rhinectomy
- 5.19.1. Introduction
- 5.19.2. Methods
- 5.19.3. Discussion
- 5.19.4. Conclusions
- Chapter 5.20. Prosthetic rehabilitation applications case study 9—Applications of 3D topography scanning and multimaterial additive manufacturing for facial prosthesis development and production
- 5.20.1. Introduction
- 5.20.2. Experimental
- 5.20.3. Results
- 5.20.4. Conclusions
- Chapter 5.21. Prosthetic rehabilitation applications case study 10—Advanced auricular prosthesis development by 3D modeling and multimaterial printing
- 5.21.1. Introduction
- 5.21.2. Experimental
- 5.21.3. Results
- 5.21.4. Conclusions
- Chapter 5.22. Prosthetic rehabilitation applications case study 11—Augmented patient-specific facial prosthesis production using medical imaging modeling and 3D printing technologies for improved patient outcomes
- 5.22.1. Introduction
- 5.22.2. Experimental
- 5.22.3. Results
- 5.22.4. Conclusions
- Chapter 5.23. Prosthetic rehabilitation applications case study 12—Customized design and development of patient-specific 3D printed whole mandible implant
- 5.23.1. Introduction
- 5.23.2. Methodology
- 5.23.3. Results
- 5.23.4. Conclusions
- Part 5.4. Orthotic applications
- Chapter 5.24. Orthotic applications case study 1—A review of existing anatomic data capture methods to support the mass customization of wrist splints
- 5.24.1. Introduction
- 5.24.2. Data acquisition methods
- 5.24.3. Contact data acquisition
- 5.24.4. Conclusion and future work
- Chapter 5.25. Orthotic applications case study 2—comparison of additive manufacturing systems for the design and fabrication of customized wrist splints
- 5.25.1. Introduction
- 5.25.2. Aim and objectives
- 5.25.3. Method
- 5.25.4. Results
- 5.25.5. Conclusions and future work
- Chapter 5.26. Orthotic applications case study 3—Evaluation of a digitized splinting approach with multiple-material functionality using additive manufacturing technologies
- 5.26.1. Introduction
- 5.26.2. Research aim and objectives
- 5.26.3. Methods
- 5.26.4. Results and discussion
- 5.26.5. Future work
- Chapter 5.27. Orthotic applications case study 4—Digitization of the splinting process: development of a CAD strategy for splint design and fabrication
- 5.27.1. Introduction
- 5.27.2. Current splinting techniques
- 5.27.3. Experimental procedures
- 5.27.4. Conclusion
- Chapter 5.28. Orthotic applications case study 5—Evaluation of a refined three-dimensional computer-aided design workflow for upper extremity splint design to support additive manufacture
- 5.28.1. Introduction
- 5.28.2. Method
- 5.28.3. Results and discussion
- 5.28.4. Conclusions and further work
- Chapter 5.29. Orthotic applications case study 6—Design optimization of a thermoplastic splint
- 5.29.1. Introduction
- 5.29.2. Original orthotic assessment
- 5.29.3. Methodology
- 5.29.4. Orthotic design
- 5.29.5. Manufacturing
- 5.29.6. Results
- 5.29.7. Manufacturing
- 5.29.8. Discussion and future work
- 5.29.9. Conclusion
- Chapter 5.30. Orthotic applications case study 7—Design and additive manufacturing of a patient-specific polymer thumb splint concept
- 5.30.1. Introduction
- 5.30.2. Existing thumb splint fabrication
- 5.30.3. Methodology
- 5.30.4. Results
- 5.30.5. Conclusion
- Chapter 5.31. Orthotic applications case study 8—Digital design and fabrication of a controlled porosity, personalized lower limb ankle foot orthosis
- 5.31.1. Introduction
- 5.31.2. Material and methods
- 5.31.3. Results and discussion
- 5.31.4. Conclusions
- Chapter 5.32. Orthotic applications case study 9—A review of 3D printed patient-specific immobilization devices in radiotherapy
- 5.32.1. Introduction
- 5.32.2. Method
- 5.32.3. Results
- 5.32.4. Discussion
- Chapter 5.33. Orthotic applications case study 10—case reports of three-dimensional printed assistive technology
- 5.33.1. Introduction
- 5.33.2. Three-dimensional printed assistive technology
- 5.33.3. Case study: Power mobility wheelchair user interface
- 5.33.4. 3D printing in mobility for recreation and leisure
- 5.33.5. Adapting the design for another user
- 5.33.6. Small design changes for a second-edition trough
- 5.33.7. Conclusion
- Part 5.5. Dental applications
- Chapter 5.34. Dental applications case study 1—the computer-aided design and additive manufacture of removable partial denture frameworks
- 5.34.1. Introduction
- 5.34.2. Materials and methods
- 5.34.3. Conclusions
- Chapter 5.35. Dental applications case study 2—trial fitting of a removable partial denture framework made using computer-aided design and additive manufacturing
- 5.35.1. Introduction
- 5.35.2. Methods
- 5.35.3. Results
- 5.35.4. Discussion
- 5.35.5. Conclusions
- Chapter 5.36. Dental applications case study 3—direct additive manufacture of removable partial denture frameworks
- 5.36.1. Introduction
- 5.36.2. Methodology
- 5.36.3. Results
- 5.36.4. Discussion
- 5.36.5. Conclusions
- Chapter 5.37. Dental applications case study 4—A comparison of plaster, digital, and reconstructed study model accuracy
- 5.37.1. Introduction
- 5.37.2. Materials and methods
- 5.37.3. Results
- 5.37.4. Discussion
- 5.37.5. Conclusions
- 5.37.6. Future work
- Contributors
- Chapter 5.38. Dental applications case study 5—Design and fabrication of a sleep apnea device using CAD/AM technologies
- 5.38.1. Introduction
- 5.38.2. Methods and materials
- 5.38.3. Results
- 5.38.4. Discussion
- 5.38.5. Conclusion
- Chapter 5.39. Dental applications case study 6—CAD/CAM/AM applications in the manufacture of dental appliances
- 5.39.1. Introduction
- 5.39.2. Material and methods
- 5.39.3. Results
- 5.39.4. Discussion
- 5.39.5. Conclusion
- Part 5.6. Research applications
- Chapter 5.40. Research applications case study 1—Bone structure models using stereolithography
- 5.40.1. Introduction
- 5.40.2. Human sample data
- 5.40.3. The use of stereolithography in the study of cancellous bone
- 5.40.4. Single human bone sample (approximately 45-mm cube)
- 5.40.5. Multiple human samples (approximately 50-mm cube)
- 5.40.6. Conclusion
- Chapter 5.41. Research applications case study 2—Recreating skin texture relief using CAD/AM
- 5.41.1. Introduction
- 5.41.2. A definition of skin texture
- 5.41.3. Identification of suitable technologies
- 5.41.4. Methods
- 5.41.5. Case studies
- 5.41.6. Results
- 5.41.7. Discussion
- 5.41.8. Conclusions
- Chapter 5.42. Research applications case study 3—Comparison of additive manufacturing materials and human tissues in computed tomography scanning
- 5.42.1. Introduction
- 5.42.2. Materials and methods
- 5.42.3. Results
- 5.42.4. Discussion
- 5.42.5. Conclusions
- Chapter 5.43. Research applications case study 4—Producing physical models from CT scans of ancient Egyptian mummies
- 5.43.1. Introduction
- 5.43.2. Technology
- 5.43.3. Case studies
- 5.43.4. Conclusions
- Chapter 5.44. Research applications case study 5—Trauma simulation of massive lower limb/pelvic injury
- 5.44.1. Introduction
- 5.44.2. Materials and methods
- 5.44.3. Discussion and conclusions
- Chapter 5.45. Research applications case study 6—biomodeling with bio-inspired soft materials
- 5.45.1. Introduction
- 5.45.2. A brief history of soft materials in medical modeling
- 5.45.3. Soft materials for medical modeling
- 5.45.4. Organic additive manufacturing
- 5.45.5. Liquid silicone rubber additive manufacturing
- 5.45.6. Craft-enhanced additive manufacturing
- 5.45.7. Characterization of analogous materials
- 5.45.8. PDMS variants
- 5.45.9. Practical applications
- 5.45.10. Conclusion
- Chapter 5.46. Research applications case study 7—Three-dimensional bone surrogates for assessing cement injection behavior in cancellous bone
- 5.46.1. Introduction
- 5.46.2. Methods
- 5.46.3. Results
- 5.46.4. Discussion
- Chapter 5.47. Research applications case study 8—Full-color medical models—Worked examples
- 5.47.1. Project background
- 5.47.2. How the project started
- 5.47.3. Project vision
- 5.47.4. Design approach
- 5.47.5. Impact on the engineering and medical professions
- 5.47.6. Future work
- Chapter 6. Future directions in medical modeling
- 6.1. Background
- 6.2. Technological advances
- 6.3. 4D printing: The next dimension in the healthcare
- 6.4. Clinical practicality at the point of care
- 6.5. Medical modeling for all
- 6.6. Summary
- Chapter 7. Glossary and explanatory notes
- 7.1. Glossary of terms and abbreviations
- 7.2. Medical explanatory notes
- Index
- No. of pages: 900
- Language: English
- Edition: 3
- Published: June 8, 2024
- Imprint: Woodhead Publishing
- Paperback ISBN: 9780323957335
- eBook ISBN: 9780323957342
RB
Richard Bibb
Prof Richard Bibb is a Professor of Medical Applications of Design at Loughborough University, UK. He graduated from Brunel University, UK (1995) with a BSc (Hons) in Industrial Design. He then undertook doctoral research in Rapid Prototyping at the National Centre for Product Design and Development Research (PDR), Cardiff Metropolitan University, UK. This study involved the development of a computerised Rapid Prototyping selection system for designers in small companies.
After gaining his PhD in 1999 he established the Medical Applications Group at PDR to conduct collaborative applied research in medical applications of design technologies such as CAD and 3D Printing. He rose to the position of Director of Research for PDR before moving to Loughborough University in 2008. In 2014 he established the Digital Design & Fabrication research lab (DDF) which focuses on advanced computer-aided design (CAD), 3D Printing and Additive Manufacturing technologies.
Professor Bibb's personal research focus is the application of advanced product design and development technologies in medicine, surgery, rehabilitation and assistive technology.
DE
Dominic Eggbeer
Prof Dominic Eggbeer is a Professor of Healthcare Applications of Design at PDR, Cardiff Metropolitan University. His research focuses on the design and development of personalized medical devices, applying his knowledge to surgical implants, facial prosthetics, dental devices and other areas of rehabilitative medicine.
In addition to his academic research, he manages a small, ISO 13485 compliant commercial team in the design of patient specific implants and other devices. Eggbeer also has a leading role in collaboration, dissemination and in supporting broad uptake of novel design engineering approaches in healthcare.
AP
Abby Paterson
Dr Abby Paterson is the Programme Director, Design for Additive Manufacturing and Lecturer in Industrial Design and Technology at Loughborough University, UK. She specialises in 3D scanning, CAD, and digital, automated fabrication (CNC milling and Additive Manufacture).
Abby graduated with a BSc in Product Design and Technology and a PhD in 3D Scanning, CAD and Additive Manufacture for Medical Applications from Loughborough University. After completing her PhD, she was appointed as a lecturer at the University of Manchester in the School of Materials; she continued her research in digital design and fabrication for medical devices and then returned to the Loughborough Design School as a lecturer in 2014.
She is currently completing a 12-month industrial fellowship in 3D scanning, CAD and AM, funded by the Royal Academy of Engineering. Abby has also received funding from Arthritis Research UK to develop specialised 3D CAD software for the design of customized 3D-printed wrist splints. In 2015, Abby was awarded a Loughborough University Teaching Innovation Award. Abby also engages with consultancy work through the Loughborough University Enterprises Ltd.
MM
Mazher Iqbal Mohammed
Dr Mazher Iqbal Mohammed is a senior lecturer in digital fabrication, a member of the Digital Design and Fabrication Research Group within the Loughborough University Design School and an Honorary Research Fellow with Deakin University’s School of Medicine.
His qualifications include a combined undergraduate Master’s in physics (MPhys) from the University of Edinburgh and an Engineering Doctorate (EngD) in medical device biotechnology from the University of Strathclyde. Dr Mohammed has worked in academia for over ten years across several institutes both in the UK and Australia, including roles at Heriot Watt University, the Australian National University (ANU) and Deakin University. During this time, he has developed a highly multidisciplinary skill set working at the intersection of design, manufacturing, material sciences, chemistry and biomedical engineering.
Dr Mohammed’s research interests focus on digitally driven fabrication technologies, aimed at positively impacting human development and longevity. He is particularly interested in the intersection of disruptive technologies such as additive manufacturing, design and data driven processes to develop innovative and practical solutions in fields ranging across healthcare, humanitarian technologies, closed-loop sustainable manufacturing and Industry 4.0.