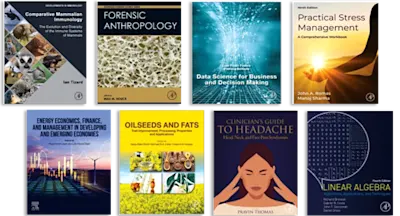
Handbook of Thin Films
- 1st Edition - October 15, 2001
- Imprint: Academic Press
- Editor: Hari Singh Nalwa
- Language: English
- Hardback ISBN:9 7 8 - 0 - 1 2 - 5 1 2 9 0 8 - 4
- eBook ISBN:9 7 8 - 0 - 0 8 - 0 5 3 3 2 4 - 7
This five-volume handbook focuses on processing techniques, characterization methods, and physical properties of thin films (thin layers of insulating, conducting, or semiconductor… Read more
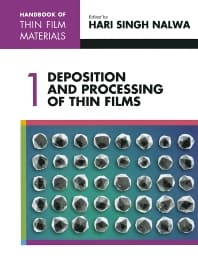
Purchase options
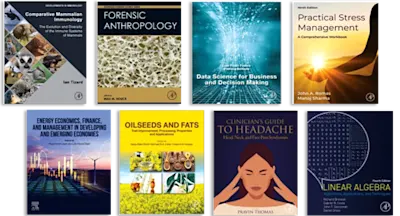
Institutional subscription on ScienceDirect
Request a sales quoteChapter 1. NANOIMPRINT TECHNIQUES
Hella-C. Scheer, Hubert Schulz, Thomas Hoffmann,
Clivia M. Sotomayor Torres
1. Introduction
1.1. Current Lithography Situation
1.2. Emerging Imprint-Based Lithography Concepts: Basic Ideas and
Expected Advantages
1.3. Overview of Imprint-Based Techniques and Nomenclature
1.4. This Chapter
2. Hot-Embossing Lithography
2.1. History of Classical Hot Embossing
2.2. Principle of Hot-Embossing Lithography
2.3. Fundamental Achievements
2.4. Imprint Systems Used for Hot Embossing
2.5. Processing Details
2.6. Viscoelastic Properties of Polymers
2.7. Fundamental Process Challenges
2.8. Hot Embossing in a Multilevel, Multilayer, or Multistep
Lithography Sequence
2.9. Room Temperature Embossing of Polymers
2.10. Self-Assembly and Wafer-Scale Embossing
2.11. Combining Hot Embossing with Other Lithography Concepts
3. Mold-Assisted Lithography
3.1. Principle of Mold-Assisted Lithography
3.2. Material Issues
3.3. Achieved Patterns
3.4. Step-and-Flash Imprint Lithography
3.5. Optical Lithography and Mold-Assisted Lithography
4. Microcontact Printing
4.1. Principle of Microcontact Printing
4.2. Material Issues
4.3. Achieved Pattems
4.4. Curved Substrates and Stamps and Large Area Printing
4.5. Related Soft-Contact Techniques
4.6. Optical Lithography with PDMS Stamps and Patterned Polymers
5. Masters, Stamps, and Molds
5.1. Master Fabrication
5.2. Replicated Stamps
5.3. Stamp Wear
6. Sticking Challenge
6.1. Physics of Adhesion
6.2. Antisticking Layers
6.3. Wear and Lifetime
7. Applications
7.1. Data Storage
7.2. Electronic Devices
7.3. Photodetectors and Light Emitters
7.4. Gratings and Integrated Optics
7.5. Biosensors
Perspectives
Acknowledgments
References
Chapter 2.
THE ENERGY GAP OF CLUSTERS, NANOPARTICLES, AND
QUANTUM DOTS
Klaus Sattler
1. Introduction
2. Experimental Techniques
2.1. Optical Spectroscopy
2.2. Scanning Tunneling Spectroscopy
3. Theory
3.1. Methods and Results for Metal Clusters
3.2. Methods and Results for Semiconductor Clusters
4. Metals
4.1. Alkali Metal Clusters
4.2. Noble and Transition Metal Clusters
4.3. Divalent Metal Clusters
5. Semiconductors
5.1. Binary Semiconductor Nanocrystals
5.2. Silicon and Germanium Clusters
6. Unpassivated Silicon Particles
6.1. Particle Preparation
6.2. Gap Measurement of Si Particles by STS
6.3. Coulomb Blockade of Si Particles
7. Passivated Silicon Particles
7.1. Pristine versus Passivated Silicon Clusters
7.2. Energy Gap Studies of H-Passivated Si Particles
8. Nanowires of Silicon
8.1. Nanowires with Various Geometries
8.2. Fullerene-Structured Si Nanowires
9. Carbon Particles
9.1. Electronic Structure of Carbon Particles
9.2. Bandgap Studies of Carbon Particles
Thin Films of Particles
10.1. Formation and Properties
10.2. Bandgap Studies of Si Particle Films
11. Applications
11.1. Photonic Devices
11.2. Cluster-Assembled Materials
11.3. Nanolithography
References
Chapter 2. 10. THE ENERGY GAP OF CLUSTERS, NANOPARTICLES, AND QUANTUM DOTS
Klaus Sattler
1. Introduction
2. Experimental Techniques
2.1. Optical Spectroscopy
2.2. Scanning Tunneling Spectroscopy
3. Theory
3.1. Methods and Results for Metal Clusters
3.2. Methods and Results for Semiconductor Clusters
4. Metals
4.1. Alkali Metal Clusters
4.2. Noble and Transition Metal Clusters
4.3. Divalent Metal Clusters
5. Semiconductors
5.1. Binary Semiconductor Nanocrystals
5.2. Silicon and Germanium Clusters
6. Unpassivated Silicon Particles
6.1. Particle Preparation
6.2. Gap Measurement of Si Particles by STS
6.3. Coulomb Blockade of Si Particles
7. Passivated Silicon Particles
7.1. Pristine versus Passivated Silicon Clusters
7.2. Energy Gap Studies of H-Passivated Si Particles
8. Nanowires of Silicon
8.1. Nanowires with Various Geometries
8.2. Fullerene-Structured Si Nanowires
9. Carbon Particles
9.1. Electronic Structure of Carbon Particles
9.2. Bandgap Studies of Carbon Particles
Thin Films of Particles
10.1. Formation and Properties
10.2. Bandgap Studies of Si Particle Films
11. Applications
11.1. Photonic Devices
11.2. Cluster-Assembled Materials
11.3. Nanolithography
References
Chapter 3. ELECTRONIC STATES IN GaAs-AIAs SHORT-PERIOD
SUPERLATTICES: ENERGY LEVELS AND SYMMETRY
Jian-Bai Xia, Weikun Ge
1. Introduction
2. Symmetry of Short-Period Superlattices
3. Photoluminescence and Photoluminescence Excitation Spectra of
Short-Period Superlattices
4. Time Decay and Temperature Dependence of Photoluminescence and Photoluminescence
Excitation Spectra
5. Photoluminescence Spectrum under Hydrostatic Pressure
6. Short-Period Superlattices in Externally Applied Fields
7. Ultra-Short-Period Superlattices
8. Other Experimental Methods and Other Oriented Short-Period Superlattices
9. Theoretical Research
10. Application of Short-Period Superlattices
11. Summary
Acknowledgment
References
Chapter 4. SPIN WAVES IN THIN FILMS, SUPERLATTICES
AND MULTILAYERS
Zhang Zhi-Dong
1. General Introduction
2. Spin Waves in Thin Films
2.1. Introduction
2.2. Macroscopic Phenomenological Theories
2.3. Quantum Microscopic Theories
3. Spin Waves in Superlattices and Multilayers
3.1. Introduction
3.2. Macroscopic Phenomenological Theories
3.3. Quantum Microscopic Theories
4. Discussion
5. Concluding Remarks
Acknowledgments
References
Chapter 5. QUANTUM WELL INTERFERENCE IN DOUBLE
QUANTUM WELLS
Zhang Zhi-Dong
1. Introduction
2. Single Quantum Wells
2.1. Introduction
2.2. Infinitely Deep Wells
2.3. Finitely Deep Eells
2.4. Effect of Vacuum
3. Mechanisms for Exchange Coupling
3.1. Introduction
3.2. Total Energy Calculations
3.3. RKKY Model
3.4. Free Electron Model
3.5. Hole Confinement Model
3.6. The Anderson Model
3.7. Quantum Well Model
4. Symmetric Double Quantum Wells: Bound States
4.1. Introduction
4.2. Model and Analytical Results
5. Symmetric Double Quantum Wells: Resonant Scattering States
5.1. Introduction
5.2. Model and Analytical Results
5.3. Numerical Results
6. Asymmetric Double Quantum Well Cu/Co/Ni/Co(100)
6.1. Introduction
6.2. Model and Analytical Results
6.3. Numerical Results
6.4. The Quantization Condition
6.5. The Special Feature of the Probabilities
7. Asymmetric Double Quantum Wells Cu/Ni/Cu/Co(100) and Cu/Co/Cu/Co(100)
7.1. Introduction
7.2. Model and Analytical Results
7.3. Numerical Results
Discussion
8.1. Advantages and Limitations of the Model
8.2. Quantum Well Effects and Exchange Coupling
8.3. Related Topics
Concluding Remarks
Acknowledgments
Appendix A
Appendix B
Appendix C
Appendix D
Appendix E
Appendix F
Appendix G
Appendix H
References
Chapter 6. ELECTRO-OPTICAL AND TRANSPORT PROPERTIES OF
QUASI-TWO-DIMENSIONAL NANOSTRUCTURED MATERIALS
Rodrigo A. Rosas, Rafd Riera, Jos6 L. Marfn, Germdn Campoy
1. Introduction
1.1. Definition of the Quasi-Two-Dimensional Nanostructured Materials
1.2. Classification of the Quasi-Two-Dimensional Nanostructured Materials
2. Methods of Synthesis and Fabrication of Quasi-Two-Dimensional
Nanostructured Materials
2.1. Molecular Beam Epitaxy
2.2. Metal-Organic Chemical Vapor Deposition
2.3. Lithography
2.4. Other Techniques
3. Electronic States of the Idealized Quasi-Two-Dimensional Nanostructured Materials
3.1. Single Heterostructure
3.2. Double Heterostructure or Single Quantum Well
3.3. Symmetric Square Double Wells: Tunneling Coupling between Wells
3.4. Superlattices
3.5. Idealized Q2D Systems when the SchrOdinger Equation that Characterizes the
Problem is Nonseparable
4. Quasi-Particle States in the Quasi-Two-Dimensional Structures
4.1. Envelope Function Approximation
4.2. Envelope Function Description of Quasi-Particle States for Q2D Systems
4.3. Excitonic States in Q2D Semiconductors
4.4. Band Structure on Realistic Q2D Nanostructures
5. Effect of Static External Electric and Magnetic Fields on the Quasi-Particle Energy Levels
in the Q2D Systems
5.1. Stark Effect in Quasi-Two-Dimensional Structures
5.2. Effect of Static External Magnetic Field on the Quasi-Particle Energy Levels in
the Q2D Structures
6. Dynamics of the Lattice in Q2D Systems: Phonons and Electron-Phonon Interactions
6.1. Lattice Oscillations
6.2. Concept of Phonons
6.3. Phenomenological Models for Long Wavelength Polar Optical Modes in
Q2D Systems
6.4. Analysis of the Phenomenological Models for Long Wavelength Polar Optical
Modes in a Semiconductor Layered System
6.5. Polaron Properties in a Semiconductor Q2D Nanostructure
7. Theory of Quantum Transport in Q2D Systems
7.1. Introduction
7.2. Electrical Conductivity in the Free Directions of Quasi-Two-Dimensional
Electron Gas
7.3. Quantum Transport in the Confinement Direction in a Quasi-Two-Dimensional
System: Vertical Transport
7.4. Magnetoconductivity of a Quasi-Two-Dimensional Electron Gas
7.5. Magnetic Field Dependence of Oxy: Quantized Hall Effect
8. Optical Properties of Q2D Nanostructured Materials
8.1. Absorption (One Electron Approximation) in Q2D Systems
8.2. Absorption: A Simplified Description of Excitonic Effects
8.3. Photoluminescence
9. Electron Raman Scattering in Q2D Systems
9.1. Model and Applied Theory
9.2. Electron Raman Scattering in a Quantum Well
9.3. Resonant Raman Scattering in Quantum Wells in High Magnetic Fields:
Fr/3hlich and Deformation Potential Interaction
10. Physical Effects of Impurity States and Atomic Systems Confined in Q2D
Nanostructured Materials
10.1. Hydrogenic Impurity in Quasi-Two Dimensional Systems
References
Chapter 7. MAGNETISM OF NANOPHASE COMPOSITE FILMS
D. J. Sellmyer, C. P Luo, Y Qiang, J. P Liu
1. Introduction and Scope
2. Nanocomposite Thin Films
2.1. Introduction
2.2. Fabrication Methods of Nanocomposite Thin Films
2.3. Theoretical Background
2.4. Structure and Properties of Magnetic Nanocomposites
3. Cluster-Assembled Thin Films
3.1. Introduction
3.2. Cluster Formation, Size Distribution, and Deposition Techniques
3.3. Cluster-Assembled Magnetic Films
3.4. Summary
4. Exchange-Coupled Nanocomposite Hard Magnetic Films
4.1. CoSm/FeCo Bilayers
4.2. Epitaxial CoSm/Fe (or Co) Multilayers
4.3. Rapid Thermally Processed Nanocomposite Films
5. Concluding Remarks
Acknowledgments
References
Chapter 8. THIN MAGNETIC FILMS
Hans Hauser, Rupert Chabicovsky, Karl Riedling
1. Magnetism Overview
1.1. Magnetic Quantities and Units
1.2. Magnetic States of Matter
1.3. Magnetic Materials for Applications
2. Magnetism of Thin Films
2.1. Magnetic Structure
2.2. Coherent Rotation of Magnetization
2.3. Surface Anisotropy and Interface Anisotropy
2.4. Exchange Anisotropy
2.5. Domain and Domain Wall Configuration
2.6. Magnetization Reversal
3. Magnetic Film Characterization
3.1. Vibrating Sample Magnetometer
3.2. Magneto-Optical Methods
3.3. Magnetic Force Microscopy
3.4. Transmission Electron Microscopy
4. Magnetic Thin Film Processing
4.1. Deposition of Magnetic Thin Films
4.2. Dry Etching of Magnetic Thin Films
5. Applications of Magnetic Thin Films
5.1. Magnetic Sensors
5.2. Magnetic Microactuators
5.3. Micro-Inductors with a Closed Ferromagnetic Core
5.4. Magnetic Data Storage
Acknowledgments
References
Chapter 9. MAGNETOTRANSPORT EFFECTS IN SEMICONDUCTORS
Nicola Pinto, Roberto Murri, Marian Nowak
Notation
1. Introduction
2. Influence of Magnetic Field on Equilibrium Carrier Density in Semiconductors
2.1. Effects Caused by High-Intensity Magnetic Fields
2.2. Fermi Level at High Magnetic Field
2.3. Fermi-Level Dependence on Impurity Concentration
2.4. Basic Equation of Charge Carrier Motion in Electric and Magnetic Fields
2.5. The Conductivity Tensor
2.6. Scattering Mechanisms of Charge Carriers in Semiconductors
2.7. Hot Electron Effects
2.8. Cyclotron Resonance
3. Hall and Galvanomagnetic Effects
3.1. Hall Effect
3.2. Galvanomagnetic Effects
3.3. Generalized Definition of the Hall Coefficients
4. Magnetoresistance
4.1. Transverse Magnetoresistance Effect
4.2. Longitudinal Magnetoresistance Effect
4.3. Behavior of Typical Semiconductors
5. Quantum Effects in Large Magnetic Fields
5.1. Shubnikov--de Haas Oscillation
5.2. Freeze-Out Effects
5.3. Magnetophonon Effect
6. Magnetotransport in Low-Dimensional Systems and in Heterostructures
6.1. Magnetotransport in Two-Dimensional Systems at Low Fields
6.2. Magnetotransport in One-Dimensional Systems at Low Fields
6.3. Low-Dimensional Systems in High Magnetic Fields
6.4. Mobility and Scattering Mechanisms in Two-Dimensional Systems
6.5. Quantized Hall Effect
7. Experimental Techniques
7.1. Resistivity of Samples with Ohmic Contacts
7.2. Galvanomagnetic Effects
7.3. Inhomogeneity and Effective Sample Thickness
7.4. The Hall Scattering Factor
7.5. Magnetoresistance and the Measure of Carder Density
7.6. The Characterization of High-Resistivity Materials
7.7. Nonuniform Material
7.8. Experimental Configurations
References
Chapter 10. THIN FILMS FOR HIGH-DENSITY MAGNETIC RECORDING
Genhua Pan
1. Instruments for Magnetic Measurement
1.1. B H and MH Loop Measurement
1.2. Magnetoresistance Measurement: Four-Point Probe Method
1.3. Schematic Frequency Permeameter
2. Basic Principles of Magnetic Recording
2.1. The Write/Read Process
2.2. Write Field of Recording Heads
2.3. Written Magnetization Transition in a Recording Medium
3. Thin Film Recording Media
3.1. Physical Limits of High-Density Recording Media
3.2. Considerations of Medium Design
3.3. Preparations of Recording Media
3.4. Characterization of Recording Media
4. Thin Films for Replay Heads
4.1. Anisotropic Magnetoresistance Films
4.2. Giant Magnetoresistance Films
4.3. Properties of Exchange-Biased Spin-Valve Films
4.4. Spin-Valve Head Engineering
5. Films for Write Heads
5.1. Basics of Soft Magnetic Films for Writers
5.2. Basics of Thin Film Writers
5.3. Magnetic Domain Configurations in Film Heads
5.4. Soft Magnetic Films for Writers
Acknowledgment
References
Chapter 11. NUCLEAR RESONANCE IN MAGNETIC THIN FILMS
AND MULTILAYERS
Mircea Serban Rogalski
1. Introduction
2. Principles of Nuclear Resonance Spectroscopy
3. Hyperline Interactions and Nuclear Resonance Spectra in Thin Solid Films
4. Experimental Techniques for Thin Film Characterization
5. Nuclear Resonance Study of Metallic Multilayers
6. Nuclear Resonance Spectroscopy in Amorphous, Nanostructured, and Granular Films
7. Concluding Remarks
Acknowledgments
References
Chapter 12. MAGNETIC CHARACTERIZATION OF SUPERCONDUCTING
THIN FILMS
M. R. Koblischka
1. Introduction
2. Local and Integral Magnetization Measurements
2.1. Other Local Techniques
2.2. Samples Used in This Study
2.3. Magneto-Optic Flux Visualization
2.4. Magnetization Measurements
2.5. AC Susceptibility Measurements
2.6. Magnetotransport
3. Theoretical Description of the Ideal Flux Patterns
3.1. Infinitely Long Strip
3.2. Other Sample Geometries
3.3. Current-Induced Flux Patterns
3.4. Magnetization Loop of a YBCO Thin Film
3.5. Flux Patterns after Field Cooling
3.6. Current Flow Reconstruction
4. Flux Patterns Around Defects and Special Magneto-Optic Experiments
4.1. Flux Creep Experiments
4.2. Visualization of Meissner Currents
4.3. Flux Patterns of a Superconducting Bend
4.4. Analysis of Flux Patterns in the Presence of Defects
4.5. Grain Boundary Studies
4.6. Large Thin Film Samples
4.7. Current Flow in Samples with Variation of Current Density
4.8. Sample for Modeling Properties of Bi-2223 Tapes
4.9. Thick Superconducting Films
4.10. Magneto-Optic Studies with High Time Resolution
5. Magnetization Measurements
5.1. Magnetization Measurements of Superconducting Thin Films
5.2. Flux Pinning in Thin Films
5.3. Virtual Additive Moments
5.4. Flux Creep Measurements
6. Conclusions
Acknowledgments
References
Index
- Edition: 1
- Published: October 15, 2001
- No. of pages (Hardback): 3451
- No. of pages (eBook): 3451
- Imprint: Academic Press
- Language: English
- Hardback ISBN: 9780125129084
- eBook ISBN: 9780080533247
HN