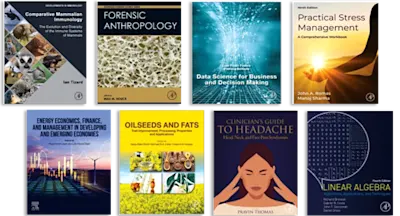
Geothermal Power Generation
Developments and Innovation
- 2nd Edition - October 11, 2024
- Imprint: Woodhead Publishing
- Editors: Ronald DiPippo, Luis Carlos Gutiérrez-Negrín, Andrew Chiasson
- Language: English
- Hardback ISBN:9 7 8 - 0 - 4 4 3 - 2 4 7 5 0 - 7
- eBook ISBN:9 7 8 - 0 - 4 4 3 - 2 4 7 5 1 - 4
Geothermal Power Generation, New Developments and Innovations, Second Edition provides an update to the advanced energy technologies that are urgently required to meet the challe… Read more
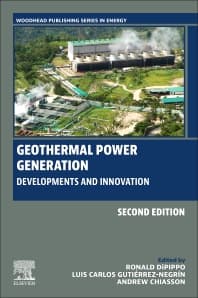
Purchase options
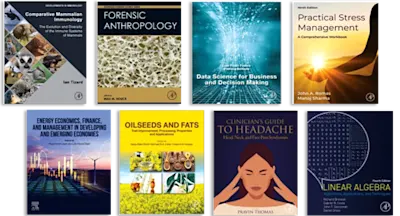
Institutional subscription on ScienceDirect
Request a sales quoteGeothermal resources are considered renewable and are currently the only renewable source able to generate baseload electricity while producing very low levels of greenhouse gas emissions, thus playing a key role in future energy needs.
- Provides readers with a comprehensive and systematic overview of geothermal power generation
- Presents an update to advanced energy technologies that are urgently required to meet the challenges of economic development, climate change mitigation, and energy security
- Edited by authorities in the field and contributed to by global experts in their areas
- Supports sustainability and the United Nations Sustainable Development Goals (UN SDGs) 7, 9, 11 and 13
- Title of Book
- Cover image
- Title page
- Table of Contents
- Copyright
- List of Contributors
- Author Biographies
- Preface to the Second Edition
- Preface to the First Edition
- 1. Introduction to Geothermal Power Generation
- 1.1 Background
- 1.2 Geothermal Resources
- 1.3 Well Drilling
- 1.4 Reservoir Engineering
- 1.5 Reservoir Monitoring
- 1.6 Engineered Geothermal Systems
- 1.7 Geothermal Power Generation
- 1.8 Geothermal as Sustainable and Renewable
- 1.9 Geothermal Reservoir Pumps
- 1.10 Geothermal Costs
- Part One. Resource Exploration, Characterization, and Evaluation
- 2. Geology of Geothermal Resources
- 2.1 Introduction
- 2.2 Heat Flow and Plate Tectonics
- 2.2.1 Installed Geothermal Electrical Generating Capacity
- 2.2.2 Geothermal Systems and Plate Tectonics
- 2.3 Temperature–Depth Relationships in Geothermal Systems
- 2.4 Geothermal Systems With Economic Potential
- 2.5 Geologic Techniques
- 2.5.1 Hydrothermal Alteration
- 2.6 Volcanic-Hosted Systems
- 2.6.1 Liquid-Dominated Geothermal Systems
- 2.6.2 Vapor-Dominated Geothermal Systems
- 2.6.3 The Geysers
- 2.7 Sediment-Hosted Geothermal Systems
- 2.8 Geothermal Systems in Extensional Tectonic Regimes
- 2.9 Unconventional Geothermal Resources
- 2.9.1 Coproduced Heat From Oil and Gas Wells
- 2.9.2 Enhanced Geothermal Systems
- 2.9.3 Closed-Loop Geothermal Systems
- 2.9.4 Supercritical Geothermal Resources
- 2.9.5 Combined Use of Geothermal Energy and Dissolved Components in Geothermal Brines
- 2.10 Conclusions
- 3. Geophysics and Resource Conceptual Models in Geothermal Exploration and Development
- 3.1 Introduction
- 3.2 Geophysics in the Context of Geothermal Decision-Making Risk Assessment
- 3.3 Geothermal Resource Conceptual Models
- 3.4 Geothermal Resource Models with Different Elements Than Those in Fig. 3.1
- 3.4.1 Detecting Magma in Geothermal Exploration
- 3.4.2 Deep Conductively Heated Stratigraphic Reservoirs
- 3.4.3 Geothermal Prospects with “Hidden” or “Blind” Reservoirs
- 3.5 Formation Properties and Geophysical Methods
- 3.6 Choosing Geophysical Methods and Designing Surveys for Geothermal Applications
- 3.7 Resistivity Methods
- 3.8 Magnetotelluric Surveys
- 3.9 Time Domain Electromagnetic Resistivity Sounding for Correction of Magnetotelluric Static Distortion
- 3.10 Awibengkok Magnetotelluric Model and Validation
- 3.11 Using Magnetotelluric Data to Build Conceptual Models and Define Resource Areas and Targets
- 3.12 Deep Low-Resistivity Zones
- 3.13 Gravity Methods for Exploration and Development
- 3.13.1 Repeat Microgravity
- 3.14 Magnetic Methods
- 3.15 Seismic Monitoring
- 3.16 Seismic Reflection/Refraction Methods
- 3.17 Borehole Wireline Logs
- 3.17.1 Borehole Image Logs
- 3.17.2 Borehole Formation Logs
- 3.17.3 Gamma Logs
- 3.17.4 Resistivity Logs and Alternative Clay Analyses
- 3.18 Self-Potential Method
- 3.19 Geophysics Management Issues
- 4. Applying Geochemistry to Resource Assessment and Geothermal Development Projects
- 4.1 Introduction
- 4.2 Early-Phase Resource Assessment
- 4.2.1 Liquid Geothermometers and Impacting Processes
- 4.2.1.1 Silica in Geothermal Fluid, Temperatures, and Mixing
- 4.2.1.2 Cation Geothermometers and Water/Rock Equilibration
- 4.2.2 Noncondensable Gas Geochemistry and Gas Geothermometers in Geothermal Systems
- 4.3 Contributions to Conceptual Models
- 4.3.1 Reservoir Temperatures
- 4.3.2 Water Sources and Recharge
- 4.3.2.1 Distinguishing Water Sources Using Stable Isotopes
- 4.3.2.2 Distinguishing Water Sources Using Gases
- 4.3.2.3 Distinguishing Geothermal Fluids and Reservoir Processes Using Water Types
- 4.3.3 Mixing
- 4.3.4 Upflow and Outflow
- 4.3.5 Reservoir Layers
- 4.3.6 Boiling
- 4.4 Geochemical Contributions to Geothermal Power Project Design
- 4.4.1 Noncondensable Gas
- 4.4.2 Potential Geothermal Emissions of Concern
- 4.4.3 Scaling
- 4.4.4 Carbonate
- 4.4.5 Silica and Silicates
- 4.4.6 Steam Purity
- 4.4.7 Corrosion
- 4.5 Geochemical Tools for Geothermal Reservoir Operation and Maintenance
- 4.6 Summary
- 5. Geothermal Well Drilling
- 5.1 Introduction
- 5.1.1 Rock Formations
- 5.1.2 High Temperature
- 5.1.3 Reservoir Pressures
- 5.1.4 Large Well and Casing Design
- 5.1.5 Well Completion Program
- 5.2 Getting Started
- 5.3 Casing Design
- 5.3.1 Grade
- 5.3.2 Tension
- 5.3.3 Burst
- 5.3.4 Collapse
- 5.3.5 Weight
- 5.4 Mud Program
- 5.5 Directional Program
- 5.6 Wellhead Design and Blowout Preventer Systems
- 5.7 Cementing Program
- 5.8 Cement Placement
- 5.8.1 Conventional Cementing
- 5.8.2 Inner String Cementing
- 5.8.3 Liner Cementing
- 5.8.4 Squeeze Cementing and Plug Cementing
- 5.8.5 Multistage Cementing
- 5.8.6 Reverse Cementing
- 5.9 Hydraulic and Bit Program
- 5.9.1 Hydraulic Program
- 5.9.2 Bits
- 5.9.2.1 Roller Cone Bits
- 5.9.2.2 Drag Bits
- 5.9.2.3 Impregnated Diamond Bits
- 5.10 Drilling Curve
- 5.11 Mud Logging
- 5.12 Drilling Rig Selection and Special Considerations
- 5.13 Cost Estimate
- 5.14 Advanced Drilling Techniques for Heat Mining
- 5.15 Concluding Summary
- 6. Characterization, Evaluation, and Interpretation of Well Data
- 6.1 Upward Convective Flow in Reservoirs
- 6.2 Pressure and Temperature Profile Analysis
- 6.2.1 Feed Zone Locations
- 6.2.2 Estimating Reservoir Pressure and Temperature
- 6.2.3 Plotting Reservoir Pressure and Temperature with Depth
- 6.2.4 Temperature Transient Analysis
- 6.3 Injection Testing
- 6.4 Discharge Tests
- 6.5 Pressure Transient Tests
- 6.5.1 Well Test Interpretation
- 6.5.1.1 Compressibility
- 6.5.1.2 Viscosity
- 6.5.2 Example
- 6.6 Wellbore Heat Loss
- 6.6.1 Single-Phase Flow
- 6.6.1.1 Production
- 6.6.1.2 Injection
- 6.6.1.3 Example Single-Phase Calculations
- 6.6.2 Two-Phase Flow
- 6.7 Summary
- 7. Reservoir Modeling and Simulation for Geothermal Resource Characterization and Evaluation
- 7.1 Review of Resource Estimation Methods
- 7.1.1 Introduction
- 7.1.2 Stored Heat Calculation
- 7.1.3 Australian Standard
- 7.1.4 Exergy
- 7.1.5 Computer Modeling
- 7.2 Computer Modeling Methodology
- 7.2.1 Basic Equations
- 7.2.2 Numerical Techniques
- 7.2.3 Equations of State
- 7.3 Computer Modeling Process
- 7.3.1 Conceptual Modeling and Computer Modeling
- 7.3.2 Interaction with Geological and Other Geoscience Models
- 7.3.3 Model Design
- 7.3.4 Boundary Conditions
- 7.3.5 Natural State, Production History, Future Scenarios
- 7.3.6 Dual Porosity versus Single Porosity Models
- 7.3.7 Model Calibration
- 7.4 Recent Modeling Experiences
- 7.4.1 Introduction
- 7.4.2 Dual Porosity
- 7.4.3 Boundary Conditions
- 7.4.4 Modeling EGS
- 7.4.5 TOUGHREACT, Scaling, and Other Chemical Processes
- 7.4.6 Carbon Dioxide in Geothermal Fields
- 7.4.7 Supercritical Geothermal Resources
- 7.5 Current Developments and Future Directions
- 7.5.1 Model Calibration
- 7.5.1.1 Long Run-times
- 7.5.1.2 Runs Fail to Finish
- 7.5.1.3 Large Number of Parameters
- 7.5.1.4 Parameter Refinement
- 7.5.1.5 Excess Enthalpy
- 7.5.2 Uncertainty Quantification Methodology
- 7.5.2.1 Systematic Errors and Inconsistencies
- 7.5.2.2 Uncertainty in Model Parameters
- 7.5.2.3 Discretization Errors
- 7.5.2.4 Measurement Errors
- 7.5.3 Application of Uncertainty Quantification to Geothermal Modeling
- 7.5.4 Data Worth Analysis
- 7.5.5 Simulator Improvements
- 7.6 Case Study of Reservoir Modeling: Lahendong, Indonesia
- 7.6.1 Background
- 7.6.2 Previous Modeling Studies
- 7.6.3 Creation of the Numerical Model
- 7.6.4 Model Calibration
- 7.6.4.1 Natural State
- 7.6.4.2 Production History
- 7.7 Conclusions
- Part Two. Energy Conversion Systems
- 8. Overview of Geothermal Energy Conversion Systems: Reservoir, Wells, Piping, Plant, and Reinjection
- 8.1 Introduction
- 8.2 It Begins with the Reservoir
- 8.3 Getting the Energy out of the Reservoir
- 8.4 Connecting the Wells to the Power Station
- 8.5 Central Power Station
- 8.6 Geofluid Disposal
- 8.7 Conclusions and a Look Ahead
- 9. Elements of Thermodynamics, Fluid Mechanics, and Heat Transfer Applied to Geothermal Energy Conversion Systems∗
- 9.1 Introduction
- 9.2 Definitions and Terminology
- 9.3 First Law of Thermodynamics for Closed Systems
- 9.4 First Law of Thermodynamics for Open Steady Systems
- 9.5 First Law of Thermodynamics for Open Unsteady Systems
- 9.6 Second Law of Thermodynamics for Closed Systems
- 9.6.1 Clausius Statement
- 9.6.2 Kelvin–Planck Statement
- 9.6.3 Clausius Inequality
- 9.6.4 Existence of Entropy
- 9.6.5 Principle of Entropy Increase
- 9.7 Second Law of Thermodynamics for Open Systems
- 9.8 Exergy and Exergy Destruction
- 9.9 Thermodynamic State Diagrams
- 9.10 Bernoulli Equation
- 9.11 Pressure Loss Calculations
- 9.12 Principles of Heat Transfer Applied to Geothermal Power Plants
- 9.13 Example Analyses for Elements of Geothermal Power Plants
- 9.13.1 Production Well
- 9.13.2 Separator
- 9.13.3 Flash Vessel
- 9.13.4 Pipelines
- 9.13.5 Turbine
- 9.13.6 Condenser
- 9.13.7 Preheater and Evaporator
- 9.14 Conclusions
- 10. Flash Steam Geothermal Energy Conversion Systems: Single-, Double-, and Triple-Flash and Combined-Cycle Plants
- 10.1 Flash Steam Cycles
- 10.1.1 Single Flash
- 10.1.2 Double and Triple Flash
- 10.1.3 Silica Scaling
- 10.1.3.1 Control of Solubility
- 10.1.3.2 Control of Precipitation Kinetics
- 10.1.3.3 Solids Separation
- 10.2 Mixed and Combined Cycles
- 10.2.1 Mixed Cycles
- 10.2.2 Combined Cycles
- 10.2.2.1 Air-Cooled Combined Cycles
- 10.2.2.2 Water-Cooled Combined Cycles
- 10.3 Cogeneration and Coproduction from Flashed Brines
- 10.3.1 Thermal Energy
- 10.3.2 Fluids
- 10.3.3 Solids
- 10.4 Equipment Research and Development
- 10.4.1 Gathering Systems and Separator Stations
- 10.4.1.1 Separator Station Design Options
- 10.4.1.2 Separator and Demister Design Options
- 10.4.1.3 Reboilers
- 10.4.1.4 Vent Stations and Turbine Bypass
- 10.4.1.5 Brine Injection Pumps
- 10.4.2 Steam Turbines
- 10.4.2.1 Condensing Steam Turbines
- 10.4.2.2 Wellhead and Back-Pressure Turbines
- 10.4.2.3 Biphase Turbines
- 10.4.2.4 Supercritical Cycles
- 10.4.3 Condensers
- 10.4.4 Cooling System Considerations for Flash Plants
- 10.4.4.1 Water-Cooled
- 10.4.4.2 Air-Cooled
- 10.4.4.3 Hybrid Cooling
- 10.4.5 Noncondensable Gas Removal Systems
- 10.4.5.1 Ejectors
- 10.4.5.2 Liquid Ring Vacuum Pumps
- 10.4.5.3 Turbocompressors
- 10.4.5.4 Other Noncondensable Gas Removal Strategies
- 10.4.6 Environmental Systems—Hydrogen Sulfide Abatement
- 10.5 Summary
- 11. Direct Steam Geothermal Energy Conversion Systems: Dry Steam and Superheated Steam Plants
- 11.1 Introduction
- 11.1.1 Early Applications
- 11.1.2 Unit Size
- 11.1.3 Power Cycle
- 11.1.4 Steam Quality
- 11.1.5 Steam Systems
- 11.1.6 Turbines
- 11.1.7 Condensers
- 11.1.8 Gas Removal Systems
- 11.1.9 Cooling Systems
- 11.1.10 Plant Auxiliaries
- 11.1.11 Engineering Materials
- 11.2 Power Cycle
- 11.2.1 Overview
- 11.2.2 Optimization of Turbine Inlet Conditions
- 11.2.3 Optimization of Turbine Exhaust Pressure and Cooling System
- 11.2.4 Optimization Around the Concentration of Noncondensable Gases
- 11.3 Steam Quality
- 11.3.1 Geothermal Steam
- 11.3.2 Vapor
- 11.3.3 Liquid and Solid Constituents
- 11.3.4 Noncondensable Gases
- 11.3.5 Chemical Constituents
- 11.4 Steam Systems
- 11.4.1 Steam Pretreatment
- 11.4.1.1 Scrubbers
- 11.4.1.2 Steam Desuperheating and Washing
- 11.4.1.3 Inertial Demisters
- 11.4.2 Steam Piping
- 11.4.2.1 Piping Material
- 11.4.2.2 Arrangement Considerations
- 11.4.2.3 Condensate Management
- 11.4.2.4 Flow Measurement Devices
- 11.5 Turbine Generators
- 11.5.1 Steam Turbines in Geothermal Service
- 11.5.1.1 Turbine Size and Configuration
- 11.5.1.2 Last-Stage Blades
- 11.5.2 Steam Path Considerations
- 11.5.2.1 Blade Profile and Shape
- 11.5.2.2 Integral Shrouds
- 11.5.2.3 Moisture Removal
- 11.5.3 Design Features for Geothermal Applications
- 11.5.3.1 Design for Scale Prevention/Mitigation
- 11.5.3.2 Moisture Limits in Turbine Exhaust Steam
- 11.5.3.3 Moisture Removal and Stage Drain Management
- 11.5.3.4 Material Enhancements for Wear Parts
- 11.5.4 Materials Selection for Turbine Internals
- 11.5.4.1 Rotors
- 11.5.4.2 Rotating Blades
- 11.5.4.3 Stationary Blades
- 11.5.4.4 Corrosion and Erosion Protection
- 11.5.5 Generators
- 11.5.5.1 Cooling
- 11.5.5.2 Corrosion Protection
- 11.5.6 Designing for Efficiency and Reliability
- 11.6 Condensers
- 11.6.1 Water-Cooled Direct-Contact Condensers
- 11.6.2 Water-Cooled Surface Condensers
- 11.6.3 Multipressure Condensers
- 11.6.4 Noncondensable Gas Cooling Zones and External Coolers
- 11.6.5 Air-Cooled Condensers
- 11.6.6 Designing for Efficiency and Reliability
- 11.7 Gas Removal Systems
- 11.7.1 Function
- 11.7.2 Steam Jet Ejectors
- 11.7.3 Ejector Condensers
- 11.7.4 Liquid Ring Vacuum Pumps
- 11.7.5 Axial or Radial Compressors
- 11.7.6 Hybrid Systems
- 11.7.7 Design for Efficiency and Reliability
- 11.7.7.1 Number of Stages
- 11.7.7.2 Stage Equipment Selection
- 11.7.7.3 Installed Spare Capacity for Rotating Equipment
- 11.7.7.4 Instrumentation
- 11.7.7.5 Motive Steam Pressure
- 11.8 Cooling Systems
- 11.8.1 Evaporative Cooling
- 11.8.2 Dry and Hybrid Cooling
- 11.8.3 Cooling Systems and Project Optimization
- 11.8.4 Cooling System Piping
- 11.9 Plant Auxiliaries
- 11.9.1 Auxiliary Cooling
- 11.9.2 Compressed Air
- 11.9.3 Fire Protection
- 11.9.4 Design Features for Geothermal Applications
- 11.10 Engineering Materials
- 11.10.1 Power Plant Materials in Geothermal Service
- 11.10.2 Steam Service
- 11.10.3 Condensate Service
- 11.10.4 Noncondensable Gas Service
- 11.10.5 Cooling Water Service
- 11.10.6 Auxiliary Services
- 11.10.7 Electrical Equipment
- 11.11 Summary
- 12. Total Flow and Other Systems Involving Two-Phase Expansion
- 12.1 Total Flow
- 12.1.1 Introduction
- 12.1.2 Previous Work
- 12.1.3 Screw Expanders
- 12.1.4 Screw Expander–Turbine Combinations
- 12.1.4.1 Single-Flash Systems
- 12.1.4.2 Double-Flash Systems
- 12.1.4.3 Parallel Screw Expander and Turbine
- 12.1.5 Turbines
- 12.1.5.1 The Biphase Turbine
- 12.1.5.2 Axial Flow Turbines
- 12.1.5.3 Radial Inflow Turbines
- 12.1.5.4 Radial Outflow (Reaction) Turbines
- 12.1.6 Conclusion
- 12.2 Alternative Systems for Power Recovery Based on Two-phase Expansion
- 12.2.1 Engineered Geothermal Systems with Water as the Power Plant Working Fluid
- 12.2.2 Binary Systems Using Organic Working Fluids
- 12.2.2.1 Binary System for Medium Enthalpy Sources
- 12.2.2.2 Trilateral Flash Cycle and Wet Organic Rankine Cycle Systems
- 12.2.3 Conclusion
- 13. Binary Geothermal Energy Conversion Systems: Basic Rankine, Dual Boiling, Supercritical, and Mixed Working Fluid Power Cycles
- 13.1 Introduction
- 13.2 Binary Power Cycle
- 13.3 Binary Cycle Performance
- 13.3.1 Performance Metrics
- 13.3.1.1 Thermal Efficiency
- 13.3.1.2 Second Law Efficiency
- 13.3.2 Efficiency Metrics Versus Power
- 13.4 Types of Binary Cycles
- 13.4.1 Subcritical Boiling Cycles
- 13.4.2 Trilateral Cycle
- 13.4.3 Supercritical Cycle
- 13.5 Selection of Working Fluid
- 13.5.1 Single-Component Working Fluids
- 13.5.2 Mixed Working Fluids
- 13.6 Geothermal Fluid Temperature Constraint
- 13.6.1 Recuperation
- 13.6.2 Turbine Extraction Feed-Heating
- 13.7 Cycle Performance Comparison
- 13.8 Design Considerations
- 13.8.1 Geothermal Heat Exchangers
- 13.8.2 Heat Rejection
- 13.8.3 Operation Under “Off-Design” Conditions
- 13.9 Economic Considerations
- 14. Combined and Hybrid Geothermal Power Systems
- 14.1 Introduction and Definitions
- 14.2 General Thermodynamic Considerations
- 14.3 Combined Single- and Double-Flash Systems
- 14.4 Combined Flash and Binary Systems
- 14.5 Geothermal–Fossil Hybrid Systems
- 14.5.1 Fossil-Fueled Superheated Geothermal Steam Plants
- 14.5.2 Coal-Fired Plants With Geothermal-Heated Feedwater
- 14.5.3 Compound Hybrid Geothermal–Fossil Plants
- 14.5.4 Gas Turbine Topped–Geothermal Flash-Steam Hybrid Plant with Superheating
- 14.5.5 Geothermal–Biomass Hybrid Plants
- 14.5.6 Geopressure Thermal–Hydraulic Hybrid Systems
- 14.6 Geothermal–Solar Hybrid Systems
- 14.6.1 Geothermal–Concentrating Solar Power Hybrid Plants
- 14.6.2 Geothermal–Photovoltaic Hybrid Plants
- 14.7 Conclusions
- Nomenclature
- 15. Enhanced Geothermal Systems: Review and Status of Research and Development
- 15.1 Introduction
- 15.2 Characterization of Geothermal Energy Systems
- 15.3 Reservoir Types Applicable for Enhanced Geothermal System Development
- 15.4 Treatments to Enhance Productivity of a Priori Low-Permeability Rocks
- 15.4.1 Thermal Stimulation
- 15.4.2 Chemical Stimulation
- 15.4.3 Hydromechanical Stimulation
- 15.4.3.1 Massive Water Injection Treatments
- 15.4.3.2 Hydraulic-Proppant Treatments
- 15.4.3.3 Multistage Treatment
- 15.5 Environmental Impact of Enhanced Geothermal System Treatments
- 15.5.1 Induced Seismicity
- 15.5.2 Measures to Mitigate Seismic Events
- 15.5.2.1 Traffic Light System
- 15.5.2.2 Cyclic Treatments
- 15.5.3 Processes with Treatment Fluids
- 15.5.4 Connecting Drinking Water Horizons
- 15.6 Sustainable Operation
- 15.6.1 Auxiliary Energy
- 15.6.2 Flow Control and Monitoring Systems
- 15.7 Closed-Loop Geothermal Systems
- 15.8 Outlook
- Part Three. Design and Economic Considerations
- 16. Waste Heat Rejection Methods in Geothermal Power Generation
- 16.1 Introduction: Overview and Scope
- 16.2 Condensers in Geothermal Power Plants
- 16.2.1 General
- 16.2.2 Impact on Thermodynamic Efficiency
- 16.2.3 Noncondensable Gases
- 16.3 Water-Cooled Condensers
- 16.3.1 General
- 16.3.2 Types of Condensers
- 16.3.2.1 Direct-Contact
- 16.3.2.2 Shell-and-Tube
- 16.3.3 Heat Dissipation
- 16.3.3.1 Once-Through Cooling Systems
- 16.3.3.2 The Cooling Tower
- 16.3.4 Operation and Maintenance
- 16.4 Air-Cooled Condensers
- 16.4.1 General
- 16.4.2 Types of Condensers
- 16.4.2.1 Direct-Contact
- 16.4.2.2 Integral Fin
- 16.4.3 Operation and Maintenance
- 16.5 Evaporative (Water- and Air-Cooled) Condensers
- 16.5.1 General
- 16.5.2 Heat Transfer Considerations
- 16.5.3 Condenser Configuration
- 16.5.4 Operation and Maintenance
- 16.6 Future Trends
- 16.7 Concluding Summary
- 17. Silica Scale Control in Geothermal Plants: Historical Perspective and Current Technology∗
- 17.1 Introduction
- 17.2 Geochemistry of Silica
- 17.3 Thermodynamics of Silica Solubility
- 17.4 Silica Precipitation Kinetics
- 17.5 Silica Scaling Experience in Geothermal Power Production
- 17.6 Historical Techniques for Silica/Silicate Scale Inhibition
- 17.7 Current Scale-Control Techniques at High Supersaturation
- 17.8 Case Study of Scale Control in Combined-Cycle Plant Design
- 17.9 Pilot-Plant Testing for Bottoming Cycle Optimization
- 17.10 Guidelines for Optimum pH-Modification System Design
- 17.10.1 Brine Flow Measurement and Control
- 17.10.2 Control System and Acid Delivery Response Time
- 17.10.3 pH Measurement
- 17.10.4 Acid Mixing and Corrosion-Resistant Materials
- 17.11 Summary
- 18. Environmental and Sociocultural Benefits and Challenges Associated With Geothermal Power Generation
- 18.1 Introduction
- 18.2 Contribution of Geothermal Energy to a Sustainable World
- 18.3 Environmental and Sociocultural Benefits and Challenges of Geothermal Projects
- 18.3.1 Sustainability Imperative
- 18.3.2 Scope of Review
- 18.3.3 Life Cycle Assessment Approach
- 18.3.4 Environmental Impacts
- 18.3.4.1 Air Quality
- 18.3.4.2 Noise
- 18.3.4.3 Geologic Impacts
- 18.3.4.4 Water Quality
- 18.3.4.5 Solid Wastes
- 18.3.4.6 Land Use
- 18.3.4.7 Forest Ecosystem and Biodiversity
- 18.3.5 Social Impacts of Geothermal Projects
- 18.3.5.1 Social Acceptance
- 18.3.5.2 Local Employment
- 18.3.5.3 Development Funds
- 18.3.5.4 Community Development Assistance
- 18.3.5.5 Involuntary Resettlement
- 18.3.6 Cultural Impacts
- 18.3.7 Summary of Benefits and Challenges of Geothermal Projects
- 18.4 Develop an Environmentally Sound and Socially Responsible Project
- 18.4.1 Conduct an Environmental Impact Assessment (EIA)
- 18.4.2 Develop a Comprehensive Environmental Management Plan
- 18.4.3 Install an Environmental Management System
- 18.4.4 Conduct a Social Impact Assessment
- 18.4.5 Facilitate Social Acceptability
- 18.4.5.1 Conduct a Stakeholder Analysis
- 18.4.5.2 Consult with Local Authorities and Communities
- 18.4.5.3 Maintain Good Relations with Stakeholders
- 18.5 Implement a Comprehensive Monitoring Program
- 18.6 Conclusions
- 19. Project Permitting, Financing, and Economics of Geothermal Power Generation
- 19.1 Introduction
- 19.1.1 Chapter Structure
- 19.1.2 Renewable Energy Finance
- 19.1.3 Power Generation and Finance
- 19.2 Financing Background
- 19.2.1 Financing Arrangements
- 19.2.2 Project Structure
- 19.3 Evidence in Geothermal Drilling and Construction
- 19.4 Costs and Financing Issues
- 19.4.1 Actors and Roles
- 19.4.2 Exploration Phase
- 19.4.3 Construction and Operational Phases
- 19.4.4 Decommissioning and Site Reclamation
- 19.5 Land Use Permitting and Interconnection
- 19.5.1 Regulatory Permit Process
- 19.5.2 Typical Project Approval Process
- 19.5.3 Involvement of Various Levels of Governance
- 19.6 Long-Term Economic and Financing Security
- 19.6.1 Risks and Risk Indemnification
- 19.6.2 Expected Risks for Merchant Plants
- 19.6.2.1 Risks and Market Participation
- 19.6.2.2 Project Risk
- 19.6.2.3 Structural Risk
- 19.6.3 Financing Tools
- 19.6.4 Financing Versus Accounting Issues
- 19.6.4.1 Accounting Standards
- 19.6.4.2 Investment Tax Credits
- 19.6.4.3 Booking Reserves
- 19.6.5 Levelized Cost of Energy and Levelized Avoided Cost of Energy
- 19.6.5.1 Limits to Levelized Cost of Energy Assumptions
- 19.6.5.2 Merchant Versus Public Projects
- 19.6.5.3 Geothermal Siting and Financial Variables
- 19.6.5.4 Well Drilling Costs
- 19.6.5.5 Financing Scenarios and Timing
- 19.6.5.6 Implied Costs of Regulation
- 19.7 Conclusions
- Appendix 19A
- 20. Mineral Recovery from Geothermal Brines
- 20.1 Introduction
- 20.2 Characteristics and Origins of Metalliferous Geothermal Fluids
- 20.3 Behavior of Dissolved Metals in Geothermal Wellbores and Separators
- 20.4 Overview of Technology for Extraction of Valuable Metals From Brines
- 20.4.1 Concentration and Precipitation
- 20.4.2 Sorption
- 20.4.3 Solvent Extraction
- 20.4.4 Membrane Separations Technology
- 20.4.5 Electrochemical Separation
- 20.5 Technology for Directly Extracting Metals from Salton Sea Geothermal Brines
- 20.6 Potential Economic Significance of Metals Recovery to Geothermal Revenue Streams
- 20.7 Conclusions
- Part Four. Case Studies
- 21. Larderello, Italy: The Oldest Geothermal Field in Operation in the World
- Prologue: Historical Outline on Geothermal Development in Italy up to 1960, with Particular Reference to the Boraciferous Region
- From Prehistory to the End of the Eighteenth Century
- The Chemical Industry of Larderello in the Nineteenth Century
- The Chemical and Geo-Power Industries from 1900 to 1960
- Concluding Remarks
- Essential References for the Prologue
- 21.1 Introduction: Background of Geothermal Power Generation
- 21.1.1 Situation in Larderello in the Late 1800s
- 21.1.2 First Attempts to Convert Thermal Energy into Mechanical Energy
- 21.2 1900–1910: First Experiments of Geo-Power Generation and Initial Applications
- 21.2.1 Rise of Ginori and New Company Policies
- 21.2.2 First Cycle that Converted Thermal into Electric Energy
- 21.2.3 Problems yet to Solve
- 21.3 1910–16: First Geothermal Power Plant of the World, Experimental Generation, and Start of Geo-Power Production at the Commercial Scale
- 21.3.1 Designing a New Plant for Production of Electricity
- 21.3.2 Producing and Selling Electricity Becomes More than a Side Business
- 21.3.3 Numerous Plant Engineering Problems Needing Solutions
- 21.4 1917–30: Consolidation of Geoelectric Power Production at the Industrial Scale and Start of a New Technology: The Direct-Cycle Geo-Power Units
- 21.4.1 Connection with the University
- 21.4.2 Increasing Retrieved Steam
- 21.4.3 New Plants to Produce Electricity are Conceived and Developed
- 21.5 1930–43: Toward a Balanced Economic Importance of Chemical Production and Geo-Power Generation
- 21.5.1 “Complete” Use of Steam
- 21.5.2 New Developments in Plant Engineering
- 21.5.3 Development of a Booming Market: Electricity for Railway Transportation
- 21.5.4 A New Company is Created
- 21.6 1944–70: Destruction, Reconstruction, Relaunching, and Modification of the Geo-Power System
- 21.6.1 Situation at the End of World War II
- 21.6.2 Larderello 3 Power Plant
- 21.6.3 New Machinery to Create and Maintain a Vacuum
- 21.6.4 Optimal Vacuum
- 21.6.5 New Types of Condensers and Gas Coolants
- 21.6.6 Power Station Arrangement
- 21.6.7 Chemical Production Decline
- 21.6.8 Travale and Mount Amiata: New Areas for Geothermal Development
- 21.6.9 New Engineering Developments
- 21.6.10 Nationalization of Electricity Production
- 21.7 1970–90: From Reinjection of Spent Fluids and Processing of Steam to the Renewal of all Power Units and Remote Control of the Whole Generation System
- 21.7.1 Reinjection of Geothermal Fluids: Initial Experiences and Results
- 21.7.2 The Oil Crises and Further Development of Geothermal Research
- 21.7.3 Standardized Production Units
- 21.7.4 Remote Control of Power Plants
- 21.7.5 Steam Washing
- 21.8 1990–2023: Recent Technological Advancements, with Special Regard to the AMIS Project, New Materials, and Environmental Acceptability
- 21.8.1 Reduction of Hydrogen Sulfide and Mercury Impact: The AMIS Project
- 21.8.2 Ammonia Abatement Plant
- 21.8.3 Visual Impact
- 21.8.4 Improved Efficiency of the Generation System
- 21.8.5 Hybrid Power Plant Technologies
- 21.8.6 Direct Uses for District Heating and Agri-Food Production
- 21.8.7 Technology Development and Sustainability
- 21.9 Other Geothermal Areas
- 21.9.1 Ischia
- 21.9.2 Latera
- 22. The Geysers: The Oldest and Largest Geothermal Power Operation in the US
- 22.1 Introduction
- 22.2 Background
- 22.3 The Fledgling Years (1960–69)
- 22.4 Geothermal Comes of Age (1969–79)
- 22.5 The Geothermal Rush (1979–86)
- 22.6 The Troubled Era (1986–95)
- 22.7 The Watershed Years (1995–98)
- 22.8 Stability at Last (1998–2004)
- 22.9 Renewed Optimism (2004–15)
- 22.10 Adapting to Climate Change and Market Forces (2015–23)
- 22.11 The Future (Beyond 2023)
- 22.12 Lessons Learned
- 23. Indonesia: Vast Geothermal Potential to Support Net Zero Emissions
- 23.1 Introduction
- 23.2 Geological Background
- 23.3 Vast Geothermal Potential
- 23.4 History of Geothermal Development in Indonesia
- 23.5 Geothermal Energy Role in the Electricity Mix
- 23.5.1 Description of the geothermal Fields in Operation
- 23.5.1.1 Gunung Salak
- 23.5.1.2 Darajat
- 23.5.1.3 Wayang Windu
- 23.5.1.4 Sarulla
- 23.5.1.5 Dieng
- 23.5.1.6 Patuha
- 23.5.1.7 Ulumbu
- 23.5.1.8 Mataloko
- 23.5.1.9 Kamojang
- 23.5.1.10 Ulubelu
- 23.5.1.11 Lahendong
- 23.5.1.12 Sibayak
- 23.5.1.13 Karaha Bodas
- 23.5.1.14 Lumut Balai
- 23.5.1.15 Sorik Marapi
- 23.5.1.16 Muara Laboh
- 23.5.1.17 Sokoria
- 23.5.1.18 Rantau Dedap
- 23.5.2 Geothermal Wells
- 23.6 Geothermal Law and Other Geothermal Regulations
- 23.6.1 Geothermal Law Number 27 of 2003 and Law Number 21 of 2014
- 23.6.2 Government Regulations (GR) No. 7 of 2017
- 23.6.3 Presidential Decree No. 4 of 2010
- 23.6.4 Pricing Policy and Presidential Regulation (PR) No. 112 of 2022
- 23.7 National Energy Condition and Policy
- 23.7.1 National Energy Condition
- 23.7.2 National Energy Policy
- 23.8 Net Zero Emissions and Energy Transition Program
- 23.9 Geothermal Development Plan
- 23.10 Future Geothermal Prospects
- 23.10.1 Hululais
- 23.10.2 Sungai Penuh
- 23.10.3 Kotamobagu
- 23.10.4 Iyang–Argopuro
- 23.10.5 Bedugul
- 23.10.6 Tulehu
- 23.10.7 Cibuni
- 23.10.8 Ciater
- 23.10.9 Rajabasa
- 23.10.10 Blawan Ijen
- 23.10.11 Jaboi
- 23.10.12 Ata Deii
- 23.10.13 Wae Sano
- 23.11 Challenges and Opportunities in Geothermal Development
- 23.11.1 The Pricing policy
- 23.11.2 Long-Term Benefit
- 23.11.3 Fiscal Incentives and FiT
- 23.11.4 Geothermal Fund
- 23.11.5 Government Guarantee
- 23.11.6 De-Risking Facilities for Geothermal Exploration
- 23.11.7 Conclusions
- 24. The Philippines: Antecedents and Perspectives of the Third Largest Geothermal Power Market
- 24.1 Introduction
- 24.2 Philippine Experience
- 24.3 General Geology
- 24.4 Distribution of Geothermal Resources
- 24.4.1 Related to Volcanic Belts
- 24.4.1.1 Santa Ana Volcanic Belt—Luzon Central Cordillera Belt—Central Luzon Belt—Cuyo Belt [16]
- 24.4.1.2 Bicol Belt—Mindanao Central Cordillera
- 24.4.1.3 Negros Belt—Sulu Belt
- 24.4.1.4 Central Mindanao Belt—Cotabato Belt
- 24.4.2 Related to Major and Other Structures [11,18]
- 24.4.3 Related to Plutonic Occurrences
- 24.5 Geothermal Fields and Power Plants
- 24.5.1 Maibarara
- 24.5.2 MakBan
- 24.5.3 Tiwi
- 24.5.4 Bacon-Manito (BacMan)
- 24.5.5 Leyte Geothermal Field Complex
- 24.5.6 Northern Negros
- 24.5.7 Negros Oriental
- 24.5.8 Mt. Apo Geothermal Field
- 24.5.9 Totals
- 24.6 The Philippine Natural Resources Framework
- 24.7 Service (Operating) Contract Under the RE Law
- 24.8 Incentives
- 24.9 Geothermal Off-Take Mechanisms
- 24.9.1 Renewable Portfolio Standards
- 24.9.2 Implementation of the Green Energy Option Program through its Integration into the Wholesale Electricity Spot Market
- 24.9.3 Inclusion of Geothermal Plants as Priority Dispatch in the Wholesale Electricity Spot Market
- 24.10 Sharing
- 24.11 Present Status and Perspectives
- 24.12 The Way Forward
- 24.13 Conclusion
- 25. Geothermal Power Generation in Central America and the Caribbean Islands
- 25.1 Introduction
- 25.2 Location, Tectonics, Seismicity, and Volcanoes
- 25.2.1 Location
- 25.2.2 Tectonics
- 25.2.3 Seismic Hazard
- 25.2.4 Volcanoes
- 25.3 Central America
- 25.3.1 General Information
- 25.3.2 Costa Rica
- 25.3.2.1 Introduction
- 25.3.2.2 Geothermal Plants in Operation
- 25.3.2.3 Future Geothermal Development and Constraints
- 25.3.3 El Salvador
- 25.3.3.1 Introduction
- 25.3.3.2 Geothermal Plants in Operation
- 25.3.3.3 Future Geothermal Development
- 25.3.4 Guatemala
- 25.3.4.1 Introduction
- 25.3.4.2 Geothermal Plants in Operation
- 25.3.4.3 Future Geothermal Development
- 25.3.5 Honduras
- 25.3.5.1 Introduction
- 25.3.5.2 Geothermal Plant in Operation
- 25.3.5.3 Future Geothermal Development
- 25.3.6 Nicaragua
- 25.3.6.1 Introduction
- 25.3.6.2 Geothermal Plants in Operation
- 25.3.6.3 Future Geothermal Development
- 25.4 Caribbean Islands
- 25.4.1 Introduction
- 25.4.2 Geothermal Plants in Operation in Guadeloupe
- 25.4.3 Future Geothermal Development
- 25.5 Final Remarks
- 26. Geothermal Power Plants at High Altitude: The Chilean Experience
- 26.1 Introduction
- 26.2 Volcano-Tectonic Setting of Chile
- 26.3 History of Geothermal Energy in Chile
- 26.4 Description of the Cerro Pabellón Geothermal Field
- 26.5 Description of the Cerro Pabellón Geothermal Reservoir
- 26.6 Cerro Pabellón Power Plant: Main Features, Wells, Electricity Generation
- 26.7 Present Status and Perspectives: Lessons Learned
- 26.8 Conclusions
- 27. Cerro Prieto, Mexico: A Large Water-Dominated Field in Operation Since 1973
- 27.1 Introduction
- 27.2 Historical Development of the Field
- 27.3 Geological Description
- 27.4 Reservoir Description and Evolution
- 27.5 Field Description
- 27.6 Status and Perspectives
- 27.7 Lessons Learned
- 28. Kenya: The Most Successful Geothermal Development in Africa
- 28.1 Introduction
- 28.2 Historical Perspectives
- 28.3 Geological Setting
- 28.3.1 Kenya Rift
- 28.3.2 Structural Setting
- 28.3.3 Petrology of the Quaternary Rocks
- 28.4 Geothermal Fields in Operation
- 28.4.1 Olkaria Geothermal Field
- 28.4.2 Eburru Geothermal Field
- 28.4.3 Menengai Geothermal Field
- 28.4.4 Paka Geothermal Prospect
- 28.4.5 Other Geothermal Prospects
- 28.5 Geothermal Power Plants in Kenya
- 28.5.1 Olkaria I Power Plant
- 28.5.2 Olkaria Wellhead Power Plants
- 28.5.3 Olkaria II Power Plant
- 28.5.4 Olkaria IV Power Plant
- 28.5.5 Olkaria V Power Plant
- 28.5.6 Oserian Power Plants
- 28.5.7 Olkaria III (OrPower4 Power Plant)
- 28.5.8 Eburru Pilot Power Plant
- 28.5.9 Menengai Geothermal Project
- 28.6 Regulatory and Institutional Framework
- 28.6.1 Institutional Framework
- 28.6.2 Regulatory Framework
- 28.6.3 Policy Framework
- 28.6.4 PPP Act No. 15 2013 (Amended 2021)
- 28.6.5 Regulation for Environmental Impact Assessment
- 28.7 Conclusions, Perspectives, and Lessons Learned
- Appendix: Worldwide Geothermal Power Plant Status-2022
- Index
- Edition: 2
- Published: October 11, 2024
- Imprint: Woodhead Publishing
- No. of pages: 958
- Language: English
- Hardback ISBN: 9780443247507
- eBook ISBN: 9780443247514
RD
Ronald DiPippo
LG
Luis Carlos Gutiérrez-Negrín
Luis Carlos Gutiérrez-Negrín is the Executive Director of the consulting company Geocónsul, SA de CV, Secretary of the CeMIE-Geo, AC, and member of the BoD of the National Institute for Electricity and Clean Energies (INEEL). He has served on the BoDs of the former GRC (now Geothermal Rising), the International Geothermal Association (IGA), and as President of the Mexican Geothermal Association. He is an Editor-in-Chief of Springer’s open-journal Geothermal Energy Science – Society – Technology and has authored/co-authored 198 peer-reviewed papers and informal communications, as well as two book chapters.
AC
Andrew Chiasson
Andrew Chiasson is an Associate Professor in the Department of Mechanical and Aerospace Engineering at the University of Dayton and is a licensed Professional Engineer in the US and Canada. He conducts research and teaches courses in the areas of Geothermal Energy, Solar Energy, Heat Transfer, Thermodynamics, and Energy Efficient Buildings. His research on geothermal energy and energy-efficient buildings has resulted in over 45 scientific publications, one book, and three book chapters.