LIMITED OFFER
Save 50% on book bundles
Immediately download your ebook while waiting for your print delivery. No promo code needed.
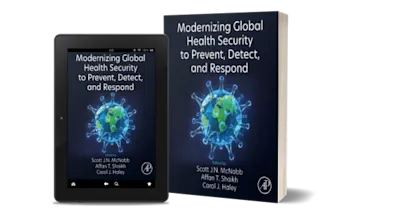
Ferrite Nanostructured Magnetic Materials: Technologies and Applications provides detailed descriptions of the physical properties of ferrite nanoparticles and thin films. Synthe… Read more
LIMITED OFFER
Immediately download your ebook while waiting for your print delivery. No promo code needed.
JP
Jitendra Pal Singh is the Ramanujan Fellow at the Manav Rachna University, Faridabad, India. His research interests are irradiation studies in nanoferrites, thin films, and magnetic multilayers, including the synthesis of ferrite nanoparticles and thin films, determining the magnetic, optical, and dielectric response of ferrites, and irradiation and implantation effects in ferrite thin films and nanoparticles.
KC
RS
OC