LIMITED OFFER
Save 50% on book bundles
Immediately download your ebook while waiting for your print delivery. No promo code needed.
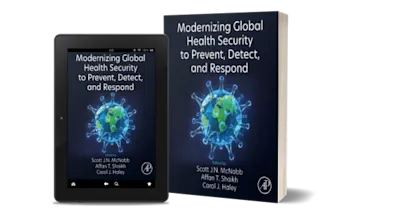
Distributed Optimal Control of Large-Scale Wind Farm Clusters: Optimal Active and Reactive Power Control, and Fault Ride Through explores the latest advances in distributed optima… Read more
LIMITED OFFER
Immediately download your ebook while waiting for your print delivery. No promo code needed.
QW
Qiuwei Wu is currently a Chair Professor at the School of Electrical and Information Engineering at Tianjin University, China. Prior to this he was a tenured Associate Professor at the Tsinghua-Berkeley Shenzhen Institute of Tsinghua University, China. His research interests are in decentralized/distributed optimal operation and control of power systems with high penetration of renewables, including distributed wind power modelling and control, decentralized/distributed congestion management, voltage control and load restoration of active distribution networks, and decentralized/distributed optimal operation of integrated energy systems. Dr. Wu is an Associate Editor of IEEE Transactions on Power Systems and IEEE Power Engineering Letters, Deputy Editor-in-Chief and Associate Editor of the International Journal of Electrical Power and Energy Systems and the Journal of Modern Power Systems and Clean Energy, and a subject editor for IET Generation, Transmission & Distribution and IET Renewable Power Generation.
SH
Sheng Huang is currently a full Professor in the College of Electrical and Information Engineering, Hunan University, China. He received his M.S. and PhD degrees both from the College of Electrical and Information Engineering, Hunan University, Changsha, China, in 2012 and 2016, respectively. His research interests are in decentralized/distributed optimal operation and coordinated control of power systems with high penetration of wind power, including renewable energy generation, modeling and integration study of wind power, and decentralized/distributed voltage control of integrated wind power systems. Prof. Huang is an Editor of IET Renewable Power Generation and Protection and Control of Modern Power Systems.
JW
Juan Wei is currently a Postdoc with the College of Electrical and Information Engineering, Hunan University, China. She received her B.S. and M.S. degrees in electrical engineering from the North China Electric Power University, Beijing, China, in 2011 and 2014, and obtained her PhD degree in electrical engineering from Hunan University, China, in 2022. Her research interests include wind power modeling and control, decentralized/distributed voltage control and optimal operation of integrated wind power systems, and high-voltage ride-through and post-event recovery control of large-scale wind farms. Dr. Wei is an Editor of Hunan Electric Power.
PW
Pengda Wang is a Research Associate with College of Electrical and Information Engineering, Hunan University, China. He obtained his PhD degree in Electrical Engineering from the Technical University of Denmark, Denmark, in 2022. His research interests lie in coordinated control of wind power and combined AC/DC grid, including distributed wind power modelling and control, voltage control and active/reactive power control of large-scale wind farms and combined AC/DC grid.
CL
Canbing Li is currently a Professor with the School of Electronic Information and Electrical Engineering, Shanghai Jiao Tong University, Shanghai, China. He received his B.E. and Ph.D. degrees from Tsinghua University, Beijing, China, in 2001 and 2006, respectively, both in electrical engineering. His research interests include power systems, smart grid, renewable energy with an emphasis on large-scale power system dispatch, economic and secure operation of power systems, energy efficiency and energy saving in smart grid, electric demand management of data centers, vehicle-to-grid technologies.
VT