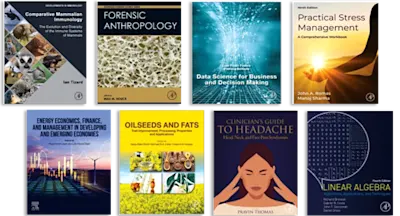
Cellular and Molecular Neurophysiology
- 5th Edition - May 30, 2024
- Imprint: Academic Press
- Author: Constance Hammond
- Language: English
- Hardback ISBN:9 7 8 - 0 - 3 2 3 - 9 8 8 1 1 - 7
- eBook ISBN:9 7 8 - 0 - 3 2 3 - 9 8 6 1 3 - 7
Unveiling the latest research in neurophysiology, Cellular and Molecular Neurophysiology, Fifth Edition continues to stand as the only resource in the field. This book remains the… Read more
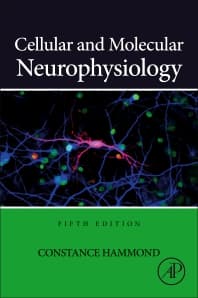
Purchase options
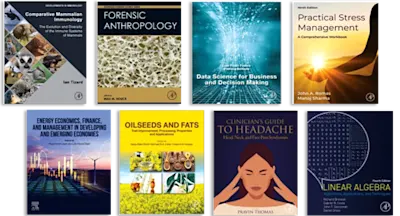
Institutional subscription on ScienceDirect
Request a sales quoteUnveiling the latest research in neurophysiology, Cellular and Molecular Neurophysiology, Fifth Edition continues to stand as the only resource in the field. This book remains the standard for those who seek to explore the intricate molecular and cellular physiology of neurons and synapses.
Notably, this new edition delves even deeper into the molecular properties and functions of excitable cells, offering unparalleled insights. In this edition, there are two groundbreaking chapters. The first reviews metabotropic receptors for olfactory transduction, while the second presents cutting-edge techniques for neuroscience research. Hypothesis-driven rather than a dry presentation of facts, the content firmly based on numerous experiments performed by top experts in the field, teaches students how to build and conduct intelligent research experiments.
This book promotes a true understanding of nerve cell function and will be a useful resource for practicing neurophysiologists, neurobiologists, neurologists, and students in a graduate-level course on the topic alike.
- Authoritative foundational coverage of basic cellular and molecular neurophysiology
- Includes new chapters on metabotropic olfactory receptors for sensory transduction and experimental techniques used by neurophysiologists
- Neurons
- Neuron-glial cell cooperation
- Ionic gradients, membrane potential, and ionic currents
- The voltage-gated channels of Na+ action potentials
- The voltage-gated channels of Ca2+ action potentials: generalization
- The chemical synapses
- Neurotransmitter release
- The ionotropic nicotinic acetylcholine receptors
- The ionotropic GABAA receptor
- The ionotropic glutamate receptors
- The metabotropic GABAB receptors
- The metabotropic glutamate receptors
- The metabotropic olfactory receptors
- Somatodendritic processing of postsynaptic potentials I: passive properties of dendrites
- Subliminal voltage-gated currents of the somatodendritic membrane
- Somatodendritic processing of postsynaptic potentials II. Role of subthreshold voltage-gated currents
- Somatodendritic processing of postsynaptic potentials III. Role of high-voltageeactivated currents
- Firing patterns of neurons
- Synaptic plasticity
- The adult hippocampal network
- Morphogenesis and maturation of the hippocampal network
- Chapter techniques
- Edition: 5
- Published: May 30, 2024
- No. of pages (Hardback): 590
- Imprint: Academic Press
- Language: English
- Hardback ISBN: 9780323988117
- eBook ISBN: 9780323986137
CH