LIMITED OFFER
Save 50% on book bundles
Immediately download your ebook while waiting for your print delivery. No promo code needed.
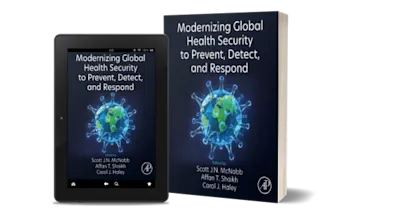
The role of environmentally triggered genetic and epigenetic changes in microbial adaptation and evolution is still not broadly appreciated. Brave Genomes: Microbial Genome Pl… Read more
LIMITED OFFER
Immediately download your ebook while waiting for your print delivery. No promo code needed.
SB
Silvia Bulgheresi is Associate Professor in Environmental Cell Biology and independent researcher at the University of Vienna. Her research on the molecular mechanisms underlying symbiont growth, division and chromosome segregation challenged long-established bacterial cell biology tenets. Since 2008, she has been teaching environmental cell biology, microbial symbioses, as well as microbial genome plasticity to Bachelor, Master ad PhD students. It is in the effort of collecting the notes, thoughts and students’ questions that arose over two decades that this book was born.