Autonomous Electric Vehicles
Nonlinear Control, Traction, and Propulsion
- 1st Edition - March 1, 2025
- Authors: Gerasimos Rigatos, Masoud Abbaszadeh, Pierluigi Siano, Patrice Wira
- Language: English
- Paperback ISBN:9 7 8 - 0 - 4 4 3 - 2 8 8 5 4 - 8
- eBook ISBN:9 7 8 - 0 - 4 4 3 - 2 8 8 5 5 - 5
Autonomous Electric Vehicles explores cutting-edge technologies revolutionizing transportation and city navigation. Novel solutions to the control problem of the complex nonlinear… Read more
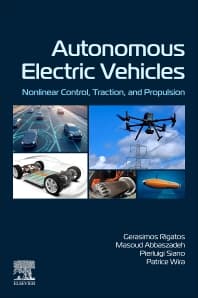
Purchase options
Institutional subscription on ScienceDirect
Request a sales quoteAutonomous Electric Vehicles explores cutting-edge technologies revolutionizing transportation and city navigation. Novel solutions to the control problem of the complex nonlinear dynamics of robotized electric vehicles are developed and tested. The new control methods are free of shortcomings met in control schemes which are based on diffeomorphisms and global linearization (complicated changes of state variables, forward and backwards state-space transformations, singularities). It is shown that such methods can be used in the steering and traction system of several types of robotized electric vehicles without needing to transform the state-space model of these systems into equivalent linearized forms. It is also shown that the new control methods can be implemented in a computationally simple manner and are also followed by global stability proofs.
- Proposes solutions for path following and localization problems of AGVs, USVs, AUVs, and UAVs, as well as solutions for the associated power supply and power management problems
- Targets jointly at improved performance for the autonomous navigation system and at optimality for the power management and electric traction system of robotized electric vehicles
- Presents nonlinear control, traction, and propulsion methods which ensure that minimization of energy consumption by autonomous electric vehicles is achieved under a zero-carbon imprint
- Is accompanied by audiovisual material explaining the contents of the individual sections of the monograph
Postgraduate students, researchers, and academics in vehicle engineering (ground, surface, underwater, aerial), intelligent transport engineering, robotics and mechatronics, electrical and computer engineering, as well as electric power systems and power electronics
Part I. Control and estimation of robotized vehicles’ dynamics and kinematics
1. Nonlinear optimal control and Lie algebra-based control
1.1 Nonlinear optimal control
1.2 Lie algebra-based control
2. Flatness-based control in successive loops for complex nonlinear dynamical systems
2.1 Flatness-based control with transformation into canonical forms
2.2. Flatness-based control implemented in successive loops
3. Nonlinear optimal control for car-like front-wheel steered autonomous ground vehicles
3.1 Kinematics/dynamics of car-like four-wheel autonomous ground vehicles
3.2 Control of the car-like vehicle using global linearization transformations
3.3 Nonlinear optimal control of car-like vehicles
3.4 Tests on path following
4. Nonlinear optimal control for skid-steered autonomous ground vehicles
4.1 Kinematics/dynamics of skid-steered autonomous ground vehicles
4.2 Control of skid-steered vehicles using global linearization transformations
4.3 Nonlinear optimal control of skid-steered autonomous ground vehicles
4.4 Tests on path following
5. Flatness-based control in successive loops for 3-DOF unmanned surface vessels
5.1 Dynamic model of a 3-DOF unmanned surface vessel
5.2 Flatness-based control in cascading loops for the 3-DOF USVs
5.3 Tests on path following
6. Flatness-based control in successive loops for 3-DOF autonomous underwater vessels
6.1 Dynamic model of a 3-DOF autonomous underwater vessel
6.2 Flatness-based control in cascading loops for 3-DOF AUVs
6.3 Tests on path following
7. Flatness-based control in successive loops for 6-DOF autonomous underwater vessels
7.1 Dynamic model of a 6-DOF unmanned underwater vessel
7.2 Flatness-based control in cascading loops for 6-DOF AUVs
7.3 Tests on path following
8. Flatness-based control in successive loops for 6-DOF autonomous quadrotors
8.1 Dynamic model of a 6-DOF autonomous quadrotor
8.2 Flatness-based control in cascading loops for 6-DOF autonomous quadrotors
8.3 Tests on path following
9. Flatness-based control in successive loops for 6-DOF autonomous octocopters
9.1 Dynamic model of a 6-DOF autonomous octocopter
9.2 Flatness-based control in cascading loops for 6-DOF autonomous octocopters
9.3 Tests on path following
10. Nonlinear optimal control for 6-DOF tilt rotor autonomous quadrotors
10.1 Dynamic model of a 6-DOF tilt-rotor autonomous quadrotor
10.2 Nonlinear optimal control of 6-DOF autonomous quadrotors
10.3. Tests on path following
11. Flatness-based adaptive neurofuzzy control of the four-wheel autonomous ground vehicles
11.1 Global linearization of the kinematic/dynamic model of a four-wheel autonomous ground vehicle
11.2 Design of a flatness-based adaptive neurofuzzy controller for four-wheel autonomous ground vehicles
11.3 Performance tests of the flatness-based adaptive neurofuzzy controller
12. H-infinity adaptive neurofuzzy control of the four-wheel autonomous ground vehicles
12.1 Approximate linearization of a kinematic/dynamic model of a four-wheel autonomous ground vehicle
12.2 Design of an H-infinity adaptive neurofuzzy controller for four-wheel autonomous ground vehicles
12.3 Performance tests of the H-infinity adaptive neurofuzzy controller
13. Fault diagnosis for four-wheel autonomous ground vehicles
13.1 Dynamic model of a four-wheel autonomous ground vehicle
13.2 Nonlinear filtering and disturbance observers for four-wheel vehicles
13.3 Statistical fault diagnosis for four-wheel autonomous vehicles
Part II. Control and estimation of electric autonomous vehicles’ traction
14. Flatness-based control in successive loops for VSI-fed three-phase permanent magnet synchronous motors
14.1 Dynamic model of a VSI-fed three-phase permanent magnet synchronous motor
14.2 Flatness-based control in cascading loops for VSI-fed three-phase permanent magnet synchronous motors
14.3 Performance tests of the flatness-based controller
15. Flatness-based control in successive loops for VSI-fed three-phase induction motors
15.1 Dynamic model of a VSI-fed three-phase induction motor
15.2 Flatness-based control in successive loops for VSI-fed 3-phase induction motors
15.3. Performance tests of the flatness-based controller
16. Flatness-based control in successive loops and nonlinear optimal control for five-phase permanent magnet synchronous motors
16.1 Dynamic model of a five-phase permanent magnet synchronous motor
16.2 Flatness-based control in successive loops for five-phase permanent magnet synchronous motors
16.3. Performance tests of the flatness-based controller
16.4 Nonlinear optimal control for five-phase permanent magnet synchronous motors
16.5. Performance tests of the nonlinear optimal controller
17. Flatness-based control in successive loops for VSI-fed six-phase asynchronous motors
17.1 Dynamic model of a VSI-fed six-phase asynchronous motor
17.2 Flatness-based control in successive loops for VSI-fed six-phase asynchronous motors
17.3. Performance tests of the flatness-based controller
18. Flatness-based control in successive lops for nine-phase permanent magnet synchronous motors
18.1 Dynamic model of a nine-phase permanent magnet synchronous motor
18.2 Flatness-based control in successive loops for nine-phase permanent magnet synchronous motors
18.3. Performance tests of the flatness-based controller
19. Flatness-based control in successive loops of a vehicle’s clutch with actuation for permanent magnet linear synchronous motors
19.1 Dynamic model of a permanent magnet linear synchronous motor-driven vehicle’s clutch
19.2 Flatness-based control in successive loops for a vehicle’s clutch
19.3. Performance tests of the flatness-based controller
20. Flatness-based control in successive loops for electrohydraulic actuators
20.1 Dynamic model of an electrohydraulic actuator
20.2 Flatness-based control in successive loops for electrohydraulic actuators
20.3. Performance tests of the flatness-based controller
21. Flatness-based control in successive loops for electropneumatic actuators
21.1 Dynamic model of an electropneumatic actuator
21.2 Flatness-based control in successive loops for electropneumatic actuators
21.3. Performance tests of the flatness-based controller
22. Flatness-based adaptive neurofuzzy control of three-phase permanent magnet synchronous motors
22.1 Global linearization of the dynamic model of a three-phase permanent magnet synchronous motor
22.2 Design of a flatness-based adaptive neurofuzzy controller for three-phase permanent magnet synchronous motors
22.3 Performance tests of the flatness-based adaptive neurofuzzy controller
23. H-infinity adaptive neurofuzzy control of three-phase permanent magnet synchronous motors
23.1 Approximate linearization of the dynamic model of a three-phase permanent magnet synchronous motor
23.2 Design of an H-infinity adaptive neurofuzzy controller for three-phase permanent magnet synchronous motors
23.3 Performance tests of the H-infinity adaptive neurofuzzy controller
24. Fault diagnosis of a hybrid electric vehicle’s powertrain
24.1 Dynamic model of a hybrid electric vehicle’s powertrain
24.2 Nonlinear filtering and disturbance observers for hybrid electric vehicles’ powertrains
24.3 Statistical fault diagnosis for hybrid electric vehicles’ powertrains
1. Nonlinear optimal control and Lie algebra-based control
1.1 Nonlinear optimal control
1.2 Lie algebra-based control
2. Flatness-based control in successive loops for complex nonlinear dynamical systems
2.1 Flatness-based control with transformation into canonical forms
2.2. Flatness-based control implemented in successive loops
3. Nonlinear optimal control for car-like front-wheel steered autonomous ground vehicles
3.1 Kinematics/dynamics of car-like four-wheel autonomous ground vehicles
3.2 Control of the car-like vehicle using global linearization transformations
3.3 Nonlinear optimal control of car-like vehicles
3.4 Tests on path following
4. Nonlinear optimal control for skid-steered autonomous ground vehicles
4.1 Kinematics/dynamics of skid-steered autonomous ground vehicles
4.2 Control of skid-steered vehicles using global linearization transformations
4.3 Nonlinear optimal control of skid-steered autonomous ground vehicles
4.4 Tests on path following
5. Flatness-based control in successive loops for 3-DOF unmanned surface vessels
5.1 Dynamic model of a 3-DOF unmanned surface vessel
5.2 Flatness-based control in cascading loops for the 3-DOF USVs
5.3 Tests on path following
6. Flatness-based control in successive loops for 3-DOF autonomous underwater vessels
6.1 Dynamic model of a 3-DOF autonomous underwater vessel
6.2 Flatness-based control in cascading loops for 3-DOF AUVs
6.3 Tests on path following
7. Flatness-based control in successive loops for 6-DOF autonomous underwater vessels
7.1 Dynamic model of a 6-DOF unmanned underwater vessel
7.2 Flatness-based control in cascading loops for 6-DOF AUVs
7.3 Tests on path following
8. Flatness-based control in successive loops for 6-DOF autonomous quadrotors
8.1 Dynamic model of a 6-DOF autonomous quadrotor
8.2 Flatness-based control in cascading loops for 6-DOF autonomous quadrotors
8.3 Tests on path following
9. Flatness-based control in successive loops for 6-DOF autonomous octocopters
9.1 Dynamic model of a 6-DOF autonomous octocopter
9.2 Flatness-based control in cascading loops for 6-DOF autonomous octocopters
9.3 Tests on path following
10. Nonlinear optimal control for 6-DOF tilt rotor autonomous quadrotors
10.1 Dynamic model of a 6-DOF tilt-rotor autonomous quadrotor
10.2 Nonlinear optimal control of 6-DOF autonomous quadrotors
10.3. Tests on path following
11. Flatness-based adaptive neurofuzzy control of the four-wheel autonomous ground vehicles
11.1 Global linearization of the kinematic/dynamic model of a four-wheel autonomous ground vehicle
11.2 Design of a flatness-based adaptive neurofuzzy controller for four-wheel autonomous ground vehicles
11.3 Performance tests of the flatness-based adaptive neurofuzzy controller
12. H-infinity adaptive neurofuzzy control of the four-wheel autonomous ground vehicles
12.1 Approximate linearization of a kinematic/dynamic model of a four-wheel autonomous ground vehicle
12.2 Design of an H-infinity adaptive neurofuzzy controller for four-wheel autonomous ground vehicles
12.3 Performance tests of the H-infinity adaptive neurofuzzy controller
13. Fault diagnosis for four-wheel autonomous ground vehicles
13.1 Dynamic model of a four-wheel autonomous ground vehicle
13.2 Nonlinear filtering and disturbance observers for four-wheel vehicles
13.3 Statistical fault diagnosis for four-wheel autonomous vehicles
Part II. Control and estimation of electric autonomous vehicles’ traction
14. Flatness-based control in successive loops for VSI-fed three-phase permanent magnet synchronous motors
14.1 Dynamic model of a VSI-fed three-phase permanent magnet synchronous motor
14.2 Flatness-based control in cascading loops for VSI-fed three-phase permanent magnet synchronous motors
14.3 Performance tests of the flatness-based controller
15. Flatness-based control in successive loops for VSI-fed three-phase induction motors
15.1 Dynamic model of a VSI-fed three-phase induction motor
15.2 Flatness-based control in successive loops for VSI-fed 3-phase induction motors
15.3. Performance tests of the flatness-based controller
16. Flatness-based control in successive loops and nonlinear optimal control for five-phase permanent magnet synchronous motors
16.1 Dynamic model of a five-phase permanent magnet synchronous motor
16.2 Flatness-based control in successive loops for five-phase permanent magnet synchronous motors
16.3. Performance tests of the flatness-based controller
16.4 Nonlinear optimal control for five-phase permanent magnet synchronous motors
16.5. Performance tests of the nonlinear optimal controller
17. Flatness-based control in successive loops for VSI-fed six-phase asynchronous motors
17.1 Dynamic model of a VSI-fed six-phase asynchronous motor
17.2 Flatness-based control in successive loops for VSI-fed six-phase asynchronous motors
17.3. Performance tests of the flatness-based controller
18. Flatness-based control in successive lops for nine-phase permanent magnet synchronous motors
18.1 Dynamic model of a nine-phase permanent magnet synchronous motor
18.2 Flatness-based control in successive loops for nine-phase permanent magnet synchronous motors
18.3. Performance tests of the flatness-based controller
19. Flatness-based control in successive loops of a vehicle’s clutch with actuation for permanent magnet linear synchronous motors
19.1 Dynamic model of a permanent magnet linear synchronous motor-driven vehicle’s clutch
19.2 Flatness-based control in successive loops for a vehicle’s clutch
19.3. Performance tests of the flatness-based controller
20. Flatness-based control in successive loops for electrohydraulic actuators
20.1 Dynamic model of an electrohydraulic actuator
20.2 Flatness-based control in successive loops for electrohydraulic actuators
20.3. Performance tests of the flatness-based controller
21. Flatness-based control in successive loops for electropneumatic actuators
21.1 Dynamic model of an electropneumatic actuator
21.2 Flatness-based control in successive loops for electropneumatic actuators
21.3. Performance tests of the flatness-based controller
22. Flatness-based adaptive neurofuzzy control of three-phase permanent magnet synchronous motors
22.1 Global linearization of the dynamic model of a three-phase permanent magnet synchronous motor
22.2 Design of a flatness-based adaptive neurofuzzy controller for three-phase permanent magnet synchronous motors
22.3 Performance tests of the flatness-based adaptive neurofuzzy controller
23. H-infinity adaptive neurofuzzy control of three-phase permanent magnet synchronous motors
23.1 Approximate linearization of the dynamic model of a three-phase permanent magnet synchronous motor
23.2 Design of an H-infinity adaptive neurofuzzy controller for three-phase permanent magnet synchronous motors
23.3 Performance tests of the H-infinity adaptive neurofuzzy controller
24. Fault diagnosis of a hybrid electric vehicle’s powertrain
24.1 Dynamic model of a hybrid electric vehicle’s powertrain
24.2 Nonlinear filtering and disturbance observers for hybrid electric vehicles’ powertrains
24.3 Statistical fault diagnosis for hybrid electric vehicles’ powertrains
- No. of pages: 400
- Language: English
- Edition: 1
- Published: March 1, 2025
- Imprint: Elsevier
- Paperback ISBN: 9780443288548
- eBook ISBN: 9780443288555
GR
Gerasimos Rigatos
Dr. Gerasimos Rigatos is Research Director of the Industrial Systems Institute, Greece, specializing in nonlinear control, nonlinear estimation, and fault diagnosis for complex dynamical systems. He has held visiting professor positions at various academic institutes and is a senior member of IEEE. He received his PhD from the National Technical University of Athens in 2000 and serves as an editor for the Journal of Information Sciences, the Journal of Advanced Robotic Systems, and the Journal of Electrified Vehicles.
Affiliations and expertise
Research Director, Unit of Industrial Automation, Industrial Systems Institute, Rion Patras, GreeceMA
Masoud Abbaszadeh
Dr. Masoud Abbaszadeh obtained his PhD in Electrical and Computer Engineering from the University of Alberta, Canada, in 2008. He has extensive experience in control systems and cyber-physical security, working at the Maplesoft–Toyota joint research team and GE Research Center. He is currently a Principal Engineer and Technical Leader of GE, while also holding an adjunct professor position at Rensselaer Polytechnic Institute. He is an associate editor of IEEE Transactions on Control Systems Technology and has over 60 patents credited to his name.
Affiliations and expertise
Principal Research Engineer, GE Global Research, Niskayuna, NY, USAPS
Pierluigi Siano
Dr. Pierluigi Siano is a Professor and the Scientific Director of the Smart Grids and Smart Cities Laboratory at the University of Salerno, Italy. His research focuses on smart grids, energy management, and power systems planning. He received his PhD from the University of Salerno in 2006 and is a distinguished visiting professor at the University of Johannesburg, South Africa. He has served as the Chair of the IES Technical Committee on Smart Grids. He is an Editor for the Power & Energy Society Section of IEEE Access, IEEE Transactions on Power Systems, IEEE Transactions on Industrial Informatics, IEEE Transactions on Industrial Electronics, and IEEE Systems.
Affiliations and expertise
Professor, Department of Management and Innovation Systems, University of Salerno, Fisciano, ItalyPW
Patrice Wira
Dr. Patrice Wira received his PhD in Electrical Engineering from Université de Haute Alsace, France, in 2002. He is a Professor at the Institut de Recherche en Informatique, Mathématiques, Automatique et Signal, Université de Haute Alsace. He specializes in artificial neural networks and adaptive control systems and their applications to power electronics. He is a senior member of IEEE and serves as an associate editor for the Energy section of the Heliyon journal (Cell Press). His research interests include control and machine learning for electric power systems and power electronics.
Affiliations and expertise
Professor, Institut de Recherche en Informatique, Mathématiques, Automatique et Signal, Université de Haute Alsace; Director, Institut Universitaire de Technologie de Mulhouse, Mulhouse, France