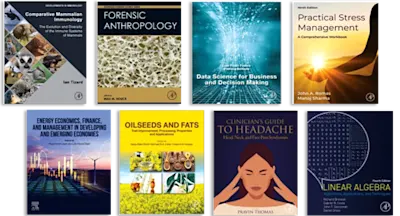
Algal Biorefinery
A Sustainable Solution for Environmental Applications
- 1st Edition - March 21, 2025
- Imprint: Elsevier
- Editors: Sanjeet Mehariya, Bikash Kumar, Shashi Kant Bhatia, Obulisamy Parthiba Karthikeyan
- Language: English
- Paperback ISBN:9 7 8 - 0 - 4 4 3 - 2 3 9 6 7 - 0
- eBook ISBN:9 7 8 - 0 - 4 4 3 - 2 3 9 6 6 - 3
Algal Biorefinery: A Sustainable Solution for Environmental Applications focuses on algae's possibilities, assets, and functions as a renewable and sustainable resource that can… Read more
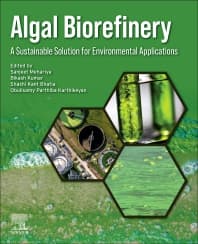
Purchase options
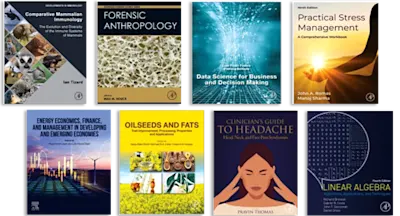
Institutional subscription on ScienceDirect
Request a sales quoteAlgal Biorefinery: A Sustainable Solution for Environmental Applications focuses on algae's possibilities, assets, and functions as a renewable and sustainable resource that can act as an excellent alternative to withstand adverse environmental conditions to generate useful products. Thus, apart from helping reduce environmental pollution and the carbon footprint, algae can help mitigate factors causing rapid climate change via concurrent bioremediation, resource recovery, and environmental sustainability.
This comprehensive book will examine dedicated state-of-the-art information on the topic of how algae can act as a cushion against climate change. It will also explain how algal-based biorefineries can act as a potential solution to climate change, lack of natural resources, and environmental pollution
- Elucidates algal biorefinery as a sustainable solution for carbon emission reduction and fossil fuels alternatives.
- Offers up-to-date information on algal-based wastewater treatment and resource recovery to assist in climate change.
- Provides flowcharts, schematic diagrams, and figures showing mechanisms and processes for the depiction of strategies for algal-based technologies.
- Examines the environmental impact assessment of existing and developing algal-based technologies for future environmental sustainability.
Provides the latest advancements in the field of algal-based technologies and applications to mitigate climate change sustainably
- Title of Book
- Cover image
- Title page
- Table of Contents
- Copyright
- Contributors
- About the editors
- Preface
- Chapter 1. Understanding carbon emission and minimizing its footprint via algal biomass-based biorefinery technologies
- 1.1 Introduction
- 1.2 The effect of carbon emission on the environment
- 1.2.1 Rising temperatures
- 1.2.2 Melting ice caps and increasing sea levels
- 1.2.3 Extreme weather events
- 1.2.4 Ecosystem disruption
- 1.2.5 Ocean acidification
- 1.3 Sources of greenhouse gas production
- 1.3.1 Burning of fossil fuels
- 1.3.2 Deforestation
- 1.3.3 Industrial processes
- 1.3.4 Agriculture
- 1.3.5 Land-use changes
- 1.4 Method of CO2 sequestration
- 1.4.1 Adsorption
- 1.4.2 Absorption
- 1.4.3 Membrane
- 1.4.4 Biological
- 1.5 Mechanism of algal biomass-based carbon sequestration
- 1.5.1 Photosynthetic process
- 1.5.2 Calvin cycle
- 1.5.3 CO2 concentrating mechanisms
- 1.6 Effect of different microalgae on CO2 biofixation
- 1.7 CO2 biofixation and biomass productivity of microalgae
- 1.7.1 Mass transfer in microalgae growth
- 1.8 Effect of CO2 concentration on microalgae growth and bubble behavior
- 1.9 Effect of reactors on microalgae growth
- 1.9.1 Open ponds
- 1.9.2 Close ponds
- 1.10 Effect of flow rate and gas velocity on microalgae growth
- 1.11 The benefit of carbon sequestration by algal biomass-based biorefinery technologies
- 1.12 Conclusion and future prospects
- Chapter 2. Algal biomass: A climate resilient feedstock for food, feed, and therapeutic applications
- 2.1 Introduction
- 2.2 Global warming
- 2.2.1 Causes of global warming
- 2.2.2 Impacts of global warming
- 2.2.3 Decline of crop productivity
- 2.3 Microalgae: A climate-resilient alternative for food, feed, and medicinal substances
- 2.4 Microalgae as a source of food
- 2.4.1 Vitamins
- 2.4.2 Minerals
- 2.4.3 Pigments
- 2.4.4 Protein
- 2.4.5 Lipids
- 2.4.6 Carbohydrates
- 2.5 Microalgae as a source of feed
- 2.5.1 Poultry feedstocks
- 2.5.2 Aquatic feedstocks
- 2.6 Microalgae as a source of therapeutic compounds
- 2.6.1 Antioxidant
- 2.6.2 Antiinflammatory
- 2.6.3 Antibacterial and antiviral
- 2.6.4 Anticancerous
- 2.7 Genetic transformation, protoplasmic fusion, and CRISPR technology in microalgae
- 2.8 Conclusion
- 2.9 Future prospects
- Chapter 3. The potential of algae from marine sources for future large-scale commercial product generation and its application
- 3.1 Introduction
- 3.2 Algal cultivation for biomass production
- 3.2.1 Open cultivation systems
- 3.2.2 Photobioreactors
- 3.2.2.1 Closed photobioreactors
- 3.2.2.2 Flat-plate photobioreactors
- 3.2.2.3 Hybrid photobioreactors
- 3.2.2.4 Tubular photobioreactors
- 3.3 Nutritional composition of algae
- 3.3.1 Lipids and fatty acids
- 3.3.2 Protein and amino acid
- 3.3.3 Carbohydrates
- 3.3.4 Vitamins
- 3.4 Use of marine algae as food
- 3.4.1 Algae for vegetarianism
- 3.5 Use of marine algae as feed
- 3.5.1 Algae as poultry feed
- 3.5.2 Algae as cattle feed
- 3.5.3 Algae as aquaculture feed
- 3.6 Algae uses for a clean environment
- 3.6.1 Role of algae in wastewater treatment
- 3.6.2 Algal response to toxic substances of industrial effluents
- 3.6.3 Algae as an alternative source of green energy
- 3.6.3.1 Bioethanol
- 3.6.3.2 Biodiesel
- 3.6.3.3 Algae a potential source of biogas
- 3.7 Economic outlook
- 3.8 Future prospect
- 3.9 Conclusion
- Chapter 4. Carbon capture by algae for sustainable product generation
- 4.1 Introduction
- 4.2 CO2 capture by algae
- 4.3 Algae as food
- 4.3.1 Valuable compounds profile of algae
- 4.3.2 Current applications in the food industry
- 4.4 Algae in animal feed and aquaculture
- 4.5 Bioactive compounds in algae: Pharmaceuticals
- 4.6 Research trend and future prospective
- 4.7 Conclusion
- AI declaration
- Chapter 5. Recovery of high value carotenoids from microalgae: Health beneficial metabolites
- 5.1 Introduction
- 5.2 Chemistry of carotenoids
- 5.2.1 Biosynthesis of carotenoids
- 5.2.2 Chemical synthesis of carotenoids
- 5.3 Carotenoids found in microalgae
- 5.3.1 β-Carotene
- 5.3.2 Astaxanthin
- 5.3.3 Lutein
- 5.3.4 Fucoxanthin
- 5.3.5 Canthaxanthin
- 5.3.6 Zeaxanthin
- 5.3.7 β-Cryptoxanthin
- 5.3.8 Lycopene
- 5.4 Extraction of carotenoids
- 5.4.1 Extraction
- 5.4.1.1 Saponification
- 5.4.1.2 Green solvent extraction
- 5.4.1.3 Enzyme-assisted extraction
- 5.4.1.4 Supercritical fluid extraction
- 5.4.1.5 Ultrasound-assisted and microwave-assisted extraction
- 5.4.1.6 Pressure-assisted and pulsed electric field-assisted extraction
- 5.4.2 Mechanisms of extraction methods
- 5.4.3 Separation, analysis, and identification
- 5.5 Application (or significance) of carotenoids to human health
- 5.5.1 Antioxidant activity
- 5.5.2 Antiinflammatory activity
- 5.5.3 Anticancer activity
- 5.5.4 Anticardiovascular activity
- 5.5.5 Neuroprotective activity
- 5.5.6 Antidiabetic activity
- 5.6 Advantages, challenges and future perspective
- 5.7 Conclusion
- Chapter 6. Algae as a potential source of biofuels and sustainable agriculture practice
- 6.1 Introduction
- 6.2 Algal biomass production
- 6.2.1 Biology and classification of algae
- 6.2.2 Algae culture conditions
- 6.2.3 Algae cultivation systems
- 6.2.4 Harvesting algae
- 6.2.5 Algal processing
- 6.3 Potential application of algae for biofuel production
- 6.3.1 Algae-based biomethane
- 6.3.2 Algae-based biohydrogen
- 6.3.3 Algae-based biodiesel
- 6.3.4 Algae-based bio-oil
- 6.4 Potential application of algae in sustainable agriculture
- 6.4.1 Algae as a source of bio-stimulants
- 6.4.2 Algae as bio-fertilizers
- 6.4.3 Algae as bio-pesticides
- 6.5 Conclusion
- Chapter 7. Unlocking the bioenergy potential of algal biomass: Microbial cell factories and innovative fermentation strategies
- 7.1 Introduction
- 7.2 Algal biorefineries—Overview
- 7.3 Pretreatment strategies
- 7.3.1 Physical treatments
- 7.3.2 Chemical treatments
- 7.3.2.1 Acid hydrolysis
- 7.3.2.2 Alkali hydrolysis
- 7.3.3 Ozonation
- 7.3.4 Ionic liquid
- 7.3.5 Other pretreatment methods
- 7.3.5.1 Ammonia fiber explosion
- 7.3.5.2 CO2 explosion/supercritical carbon dioxide
- 7.3.6 Biological methods
- 7.4 Enzyme saccharification
- 7.4.1 Axenic hydrolytic cultures
- 7.4.2 Bacterial consortia
- 7.4.3 Consolidated bioprocesses
- 7.5 Biorefinery of algal biomass
- 7.5.1 Biohydrogen
- 7.5.2 Biodiesel
- 7.5.3 Bioethanol
- 7.5.4 Organic acids and biopolymers
- 7.6 Life-cycle assessment and techno-economic analysis
- 7.7 Challenges and limitations of algal biorefinery
- 7.8 Conclusion
- Chapter 8. Cultivation of microalgae on anaerobic digestate: Case studies
- 8.1 Introduction
- 8.2 Cultivation of microalgae on digestate
- 8.2.1 Digestate characteristics and requirements for cultivation
- 8.2.2 Cultivating microalgae on digestate
- 8.3 Case studies
- 8.3.1 Case Study 1: ALG-AD
- 8.3.2 Case Study 2: AlgaeBioGas
- 8.4 Processing and utilization of the biomass for feed, bioenergy, and biostimulants
- 8.4.1 Biorefineries
- 8.5 Trends, challenges, and opportunities
- 8.5.1 Market and research trends
- 8.5.2 Challenges and opportunities
- 8.5.2.1 Costs
- 8.5.2.2 Regulation and legislation
- 8.6 Conclusion
- Chapter 9. Decarbonizing the oil and gas industry via algal biorefinery: Drivers and barriers analysis using q-rung orthopair fuzzy DEMATEL approach
- 9.1 Introduction
- 9.1.1 Literature review
- 9.2 The decision-making framework
- 9.2.1 The drivers and barriers
- 9.2.2 Different techniques/approaches for algal biorefinery
- 9.2.3 The DEMATEL method based on the q-ROFS
- 9.2.3.1 The q-ROFS
- 9.2.3.2 Procedure of DEMATEL technique under the q-ROFS
- 9.2.4 The results related to the q-ROFS-based DEMATEL technique
- 9.3 Conclusions and future prospects
- Chapter 10. Algal technologies in waste treatment and environmental remediation
- 10.1 Introduction
- 10.2 Wastewater typology
- 10.2.1 Mine wastewater
- 10.2.2 Pharmaceutical and personal care products from urban effluents
- 10.2.3 Pollutants from agroindustry
- 10.2.4 Industrial wastewater treatment
- 10.3 Microalgae as a bioremediation tool
- 10.3.1 Main challenges in wastewater treatment by microalgae
- 10.3.2 Critical factors to bioremediation efficiency
- 10.4 Circular bioeconomy approach
- 10.4.1 Biomass collection
- 10.4.2 Reducing algal growth problems
- 10.4.3 Potentially toxic element problem
- 10.4.4 Prospects in a changing world
- 10.5 Conclusion
- Chapter 11. Microalgae-mediated pollutant removal from wastes of pharmaceutical and personal care products industry—Current trends
- 11.1 Introduction
- 11.2 Pharmaceutical and personal care products as emerging pollutants
- 11.2.1 Sources of pharmaceutical and personal care products contaminants
- 11.2.2 Routes of pharmaceutical and personal care products contaminants
- 11.2.3 Concerns over pharmaceutical and personal care products contaminants
- 11.2.4 Pharmaceutical components
- 11.2.4.1 Antibiotics
- 11.2.4.2 Analgesics and antiinflammatory drugs
- 11.2.4.3 Antidepressants
- 11.2.4.4 Steroid hormones
- 11.2.5 Cosmetics
- 11.2.5.1 Parabens
- 11.2.5.2 Phthalates
- 11.2.5.3 Sulfates
- 11.2.5.4 Phenols
- 11.2.5.5 Plastic microbeads
- 11.2.5.6 Ultraviolet filters
- 11.3 Mechanisms of pharmaceutical and personal care products removal by microalgae
- 11.3.1 Metabolic pathways
- 11.3.1.1 Bioadsorption
- 11.3.1.2 Bioaccumulation
- 11.3.1.3 Biodegradation
- 11.3.1.4 Photobiodegradation
- 11.3.1.5 Volatilization
- 11.3.2 Nonmetabolic pathways
- 11.3.3 Cometabolism
- 11.4 Factors influencing the pharmaceutical and personal care products removal
- 11.4.1 Temperature
- 11.4.2 Hydraulic retention time
- 11.4.3 Light intensity
- 11.4.4 Phylogenetic diversity of microalgae
- 11.4.5 Toxicity of pharmaceutical and personal care products on microalgae
- 11.4.6 Nutrient deficiency and pH
- 11.5 Microalgae in pharmaceutical and personal care products removal
- 11.5.1 Axenic strains
- 11.5.2 Consortial banks
- 11.5.3 Genetically engineered microalgal strains
- 11.5.4 Acclimatization and adaptive laboratory evolution of microalgal strains
- 11.5.5 Microalgae-derived enzymes
- 11.5.6 Microalgae-derived biochar
- 11.6 Microalgal reactors for phycoremediation
- 11.6.1 Suspended systems
- 11.6.1.1 Open system
- 11.6.1.2 Enclosed system
- 11.6.2 Immobilized systems
- 11.6.2.1 Matrix encapsulation-based immobilization systems
- 11.6.2.2 Biofilm-based immobilization
- 11.6.3 Hybrid systems
- 11.6.3.1 Semiclosed system
- 11.7 Integrated approaches in pharmaceutical and personal care products pollutant removal
- 11.7.1 Algal–bacterial integrated approach
- 11.7.2 Algal–fungal integrated approach
- 11.7.3 Algal–nanotechnology integrated approach
- 11.7.3.1 Effect of pH and other factors on algal-synthesized nanoparticles in pharmaceutical and personal care products removal
- 11.7.4 Algal–co-substrate integrated approach
- 11.7.5 Phycoremediation – other integrated approaches
- 11.8 Bioeconomy in phycoremediation of pharmaceutical and personal care products
- 11.9 Environmental risk assessment of microalgae-mediated pharmaceutical and personal care products pollutant removal
- 11.10 Future perspectives
- 11.11 Conclusion
- Chapter 12. Biotechnological advances for wastewater treatment and resource recovery using algae and cyanobacteria
- 12.1 Introduction
- 12.2 Wastewater treatment using algae and cyanobacteria
- 12.2.1 Potential of microalgae and cyanobacteria for wastewater remediation
- 12.2.2 Nutrient removal
- 12.2.3 Heavy metals removal
- 12.2.4 Persistent organic pollutants removal
- 12.2.5 Microplastics removal
- 12.2.6 Algal–bacterial interactions in wastewater treatment
- 12.3 Algae and cyanobacteria-based treatment systems
- 12.3.1 Application of microalgae and cyanobacteria in the treatment of domestic and industrial wastewater
- 12.3.2 Open ponds systems
- 12.3.3 Closed photobioreactors
- 12.4 Resource recovery from algae and cyanobacteria grown in wastewater
- 12.4.1 Nutrients recovery
- 12.4.2 Biomolecules recovery
- 12.4.3 Biopolymers recovery
- 12.5 Challenges and opportunities of omics application in the bioremediation of wastewater and resource recovery using algae and cyanobacteria
- AI declaration
- Chapter 13. Role of algal-based technologies in domestic wastewater treatment for resources recovery and water reuse
- 13.1 Introduction
- 13.2 Phycoremediation of domestic wastewater by microalgae at laboratory scale
- 13.3 Technologies-based microalgae in wastewater treatment—Pilot scale
- 13.4 Disinfection process of wastewater by microalgae culture
- 13.5 Installation potential of wastewater phycoremediation process in rural areas
- 13.6 Possible wastewater reuse from phycoremediation processes
- 13.6.1 Urban reuse
- 13.6.2 Agriculture reuse
- 13.6.3 Construction
- 13.6.4 Phycoremediation of wastewater as an integrated resource system
- 13.7 Subproducts production from microalgae biomass obtained in wastewater treatments
- 13.7.1 Biofertilizers
- 13.7.2 Metabolites
- 13.7.2.1 Fatty acids
- 13.7.2.2 Pigments
- 13.7.2.3 Polysaccharides
- 13.7.3 Biofuels
- 13.7.4 Food source
- 13.7.5 Bioplastics
- 13.8 Future perspectives
- 13.9 Conclusions
- 13.10 Summary points
- Chapter 14. Wastewater treatment employing microbial carbon-capture cells with concomitant bioenergy production and valuable product recovery
- 14.1 Introduction
- 14.2 MCC
- 14.3 Applications of microbial carbon-capture cell
- 14.3.1 Carbon capture
- 14.3.2 Wastewater treatment and electricity generation
- 14.3.3 Valuable production
- 14.4 Other carbon-capturing bioelectrochemical systems
- 14.5 Advantage of MCC over other carbon-capturing bioelectrochemical systems
- 14.6 Factors affecting the performance of microbial carbon-capture cell
- 14.7 Bottlenecks and perspectives in scaling-up of microbial carbon-capture cell
- 14.8 Conclusion
- Chapter 15. Can algal based technologies help achieve zero liquid discharge (ZLD) in the industrial sector?
- 15.1 Introduction
- 15.1.1 What is ZLD and why is it needed?
- 15.1.2 Challenges in achieving zero liquid discharge
- 15.1.2.1 Intensive energy consumption
- 15.1.2.2 High capital and operational costs
- 15.1.2.3 Land requirements
- 15.1.2.4 Greenhouse gas emissions
- 15.1.2.5 Complex brine composition
- 15.1.3 Incentives for industries to adopt algal-based treatment to achieve ZLD
- 15.2 Identifying potentials of algae-based technologies in industrial wastewater treatment
- 15.2.1 Energy conservation
- 15.2.2 Carbon sequestration
- 15.2.3 Complex wastewater management
- 15.3 Integration of algal-based treatments into industrial wastewater treatment
- 15.3.1 Retrofitting into existing treatment systems
- 15.3.2 Designing industry-specific treatment systems
- 15.3.2.1 Treatment of highly alkaline winery wastewater
- 15.3.2.2 Chromium remediation from electroplating wastewater
- 15.3.2.3 Algae-bacterial consortia for industrial wastewater treatment
- 15.4 Key findings and future prospects
- 15.4.1 Key findings on potential and challenges
- 15.4.2 Prospects for future research
- 15.5 Conclusion
- Chapter 16. Advances in technologies for biotransformation of algal biomass into high-value products
- 16.1 Introduction
- 16.2 Advances in technologies for biotransformation of algal biomass
- 16.2.1 Physical technologies
- 16.2.1.1 Thermal pretreatment
- 16.2.1.2 Mechanical pretreatments
- 16.2.2 Algal cell disruption
- 16.2.2.1 Microfluidic devices
- 16.2.2.2 Electroporation
- 16.2.3 Algae dewatering and concentration
- 16.2.3.1 Acoustic wave separation
- 16.2.3.2 Dielectrophoresis
- 16.2.4 Physico-chemical technologies
- 16.2.4.1 Hydrothermal liquefaction (HTL)
- 16.2.4.2 Supercritical fluid extraction (SFE)
- 16.2.5 Biological technologies
- 16.2.5.1 Microbial consortia
- 16.2.5.2 Genetic engineering of algae
- 16.2.5.3 Enzymatic conversion
- 16.2.5.4 Algae-microbe synergy: Maximizing resource efficiency
- 16.3 High-value products from algal biomass and their market demand
- 16.3.1 Pharmaceuticals
- 16.3.2 Nutraceutical
- 16.3.3 Cosmeceutical
- 16.4 Current trend and future prospective
- 16.4.1 Enhanced algal strain development
- 16.4.2 Integration of multiomics approaches
- 16.4.3 Algal biorefineries at scale
- 16.4.4 Algae-based carbon capture and utilization (CCU)
- 16.4.5 Algae for sustainable agriculture
- 16.4.6 Regulatory and environmental considerations
- 16.5 Conclusion
- Chapter 17. Bottlenecks in applications of algal-based technologies toward industrial commercialization
- 17.1 Introduction
- 17.2 Technical and operational bottlenecks
- 17.2.1 Strain selection
- 17.2.2 Scale-up and photobioreactor designs of the cultivation systems
- 17.2.3 Harvesting and dewatering
- 17.2.4 Extraction and purification
- 17.3 Economic, market, and regulatory bottlenecks
- 17.3.1 Economic viability and cost
- 17.3.2 Market and commercialization strategies
- 17.3.3 Research and development funding
- 17.3.4 Regulatory and compliance issues
- 17.3.5 Public perception and acceptance
- 17.4 Environmental and sustainable bottlenecks
- 17.4.1 Land and water requirement
- 17.4.2 Nutrient pollution and eutrophication
- 17.4.3 Invasive species and biosecurity
- 17.5 Emerging solutions and innovations of industrial commercialization of algal-based technologies
- 17.6 Future prospects
- 17.7 Conclusion
- Chapter 18. Bottleneck and opportunities toward practical aspects of microalgae-based biorefineries
- 18.1 Introduction
- 18.2 Biorefinery of microalgae
- 18.2.1 Transesterification
- 18.2.2 Microalgal biomass biochemical conversion for biofuel production
- 18.2.2.1 Bioethanol production
- 18.2.2.2 Biogas production
- 18.2.2.3 Biohydrogen production
- 18.3 Bottlenecks in microalgal biorefineries
- 18.4 Opportunities toward microalgae-based biorefineries
- 18.5 Conclusions
- Chapter 19. Life cycle assessment and techno-economic sustainability assessment of existing algal-based technologies for future implications
- 19.1 Introduction
- 19.2 Microalgae biorefinery technologies
- 19.3 Biorefinery sustainability
- 19.3.1 Scientific advances of algal biorefinery sustainability assessment
- 19.3.2 LCA methodologies challenges
- 19.3.2.1 Functional unit (FU)
- 19.3.2.2 Boundary conditions
- 19.3.2.3 Allocation
- 19.3.2.4 Sensitive and uncertainty analysis
- 19.3.2.5 Data collection
- 19.4 LCA applied to algal biorefineries
- 19.5 Future of the industry
- 19.5.1 Ecosystem service and carbon capture
- 19.5.2 LCA and TEA methodologies
- 19.5.3 Market, standards, and legislation
- 19.6 Conclusions
- Chapter 20. Immobilized microalgae cultivation systems: Pros and cons concerning environmental impact assessment (EIA)
- 20.1 Introduction
- 20.2 Environmental impact assessment (EIA)—A need for sustenance
- 20.3 Algal cultivation systems
- 20.3.1 Suspended systems
- 20.3.2 Immobilized systems
- 20.3.3 Hybrid systems
- 20.4 EIA of algal cultivation systems
- 20.4.1 Water and its impact
- 20.4.2 Land use
- 20.4.3 Nutrients and fertilizers and their ecological impacts
- 20.4.4 CO2 sequestration
- 20.4.5 Fossil fuel/electricity inputs
- 20.4.6 Genetically modified algae and their impacts
- 20.4.7 Algal toxicity and gaseous exchange
- 20.4.8 Energy balance
- 20.5 Microalgae harvesting technologies and their environmental impact
- 20.5.1 Scraping, squeezing, interfacial interaction, and spooling in immobilized systems
- 20.5.2 Dewatering
- 20.6 Future prospects
- 20.7 Conclusion
- Chapter 21. Future prospect of development of integrated wastewater and algal biorefineries and its impact on biodiversity and environment
- 21.1 Introduction
- 21.2 General aspects of microalgae
- 21.3 Bioremediation in synergy with microalgae: Effluent treatment
- 21.4 Main mechanisms used in bioremediation
- 21.4.1 Biosorption
- 21.4.2 Bioaccumulation
- 21.4.3 Biodegradation
- 21.5 Impacts on biodiversity
- 21.6 Economic impacts
- 21.7 Future perspectives
- 21.8 Conclusion
- AI declaration
- Index
- Edition: 1
- Published: March 21, 2025
- No. of pages (Paperback): 412
- No. of pages (eBook): 408
- Imprint: Elsevier
- Language: English
- Paperback ISBN: 9780443239670
- eBook ISBN: 9780443239663
SM
Sanjeet Mehariya
BK
Bikash Kumar
Dr. Bikash Kumar is currently working as a National Post-Doctoral Fellow, at the Indian Institute of Technology, Indore. His work is focused on the development of biomass pretreatment technologies, and microbial enzyme production (cellulases, laccase, xylanases) for greener energy and environmental sustainability. He has worked as a Post-Doctoral Research Associate at the Department of Biosciences and Bioengineering at the Indian Institute of Guwahati, Guwahati Assam. Dr. Kumar earned his PhD from the Department of Microbiology, Central University of Rajasthan, India. The work has resulted in the publication of more than 21+ research/review papers, and 23+ book chapters. He has Edited a book on radiation-based technologies for biorefinery applications for Springer and has been acknowledged for Editorial assistance to Prof. Pradeep Verma in compiling 11 books for CRC Press, Elsevier, and Springer. His work has attracted significant impact which is evident from 2300+ citations with h-index – 23, & i-10-index-27. He is the recipient of several awards and Fellowships such as the AMI-Young Scientist Award, INSA-NASI-SRF, CSIR-SRF, JNMF scholarship, etc. He is a life member of the Biotech Research Society of India (BRSI); the Association of Microbiologists of India (AMI); the Mycological Society of India (MSI) and the Association of Carbohydrate Chemists and Technologists, India (ACCTI). He is a reviewer of 20+ peer-reviewed research journals for Elsevier, Wiley, MDPI, Springer, Taylor, and Francis. Dr. Bikash Kumar is working as Associate Editor in Frontiers in Energy Research, Guest Editor for a Special Issue in Frontiers in Bioengineering and Biotechnology (Green Synthesis of Catalyst for Sustainable Industrial Technologies), and Review Editor for Frontiers in Chemistry. Dr. Kumar is the recipient of DST-SERB NPDF project funding of 2.711 million INR. He is included in the top 2% of the Scientist List at Stanford University in the list for year 2023.
SB
Shashi Kant Bhatia
Dr. Shashi Kant Bhatia is an Associate professor in the Department of Biological Engineering, Konkuk University, Seoul, South Korea, and has more than ten years’ experience in biowastes valorization into bioenergy, biochemicals, and biomaterials. He holds an MSc and a PhD in biotechnology at Himachal Pradesh University (India). Dr. Bhatia has worked as a Brain Pool Post Doc Fellow at Konkuk University (2014–2016) and has contributed extensively to the industrial press and served as an editorial board member of Sustainability and Energies journal and associate editor of Frontier of Microbiology, Microbial Cell Factories, Biomass Conversion and Biorefinery, Bioprocess and Biosystem Engineering, Carbohydrate Technology and Applications, 3 Biotech, and PLOS One journals. He is editor in Chief of Biotechnology for Sustainable Materials journal. He has published more than 200 research and review articles on industrial biotechnology, bioenergy production, biomaterial, biotransformation, microbial fermentation, and enzyme technology in international scientific peer-reviewed journals and holds 15 international patents. He has also edited 7 books on microbial biotechnology. With more than 12000 citations with an h-index of 60 and an i10 index of 195 (As per Google Scholar), he is included in the top 2% of the Scientist List at Stanford University. Dr. Bhatia has successfully supervised and completed three projects funded by NRF Korea ($ 600, 000).
OK
Obulisamy Parthiba Karthikeyan
Dr. Obulisamy Parthiba Karthikeyan (OPK) is a Director of EHS at Austin Elements Inc., Houston, Texas, USA. He has over 15 years of international research experience in the field of environmental microbiology and biotechnology. He had secured a sum of $500,000 of research/consultancy funding within the last 8 years from national/international agencies. He is nominated for the prestigious Presidential Chinese Academy of Science Awards 2021 from Institute of Subtropical Agriculture, Changsha, China. In the past, he had achieved few meritorious awards such as Australia-Thailand Early Career Research Exchange Award-2014, Sêr Cymru National Research Network for Low Carbon Energy and Environment Writing Fellowship and Award-2018 (UK), and Ramalingaswamy Re-Entry Fellowship-2018 (India).
Dr. OPK is serving as a peer review member assessor for the United States Department of Agriculture (USDA)-USA, Agriculture and Agri-Food Canada, Canada, Horizon H2020-Marie Sklodowska-Curie Individual Fellowships-Europe, Australian Research Council-Australia, and the Research Foundation – Flanders (FWO)- Belgium. He is associated editor for the Frontiers of Microbiotechnology and guest edited few special issues for few publishers such as Elsevier, Springer and MDPI. He has published more than 75 research/review articles in Scopus indexed journals, 4 books, 12 chapters and 4 editorials. His research interests and knowledge spans on various research topics including microbial engineering, omics for process understanding, Greenhouse gas fermentation into value products, waste biorefinery, Bioreactor engineering and Bioleaching of metals.