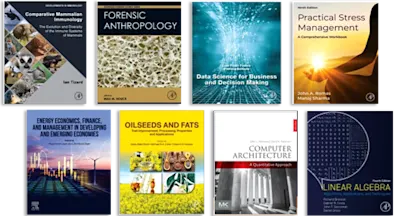
Aerodynamics for Engineering Students
- 8th Edition - November 22, 2024
- Imprint: Butterworth-Heinemann
- Authors: Steven H. Collicott, Daniel T. Valentine, E. L. Houghton, P. W. Carpenter
- Language: English
- Paperback ISBN:9 7 8 - 0 - 3 2 3 - 9 9 5 4 4 - 3
- eBook ISBN:9 7 8 - 0 - 3 2 3 - 9 5 8 1 5 - 8
Aerodynamics for Engineering Students, Eight Edition provides concise explanations of basic concepts combined with an excellent introduction to aerodynamic theory. This updated e… Read more
Purchase options
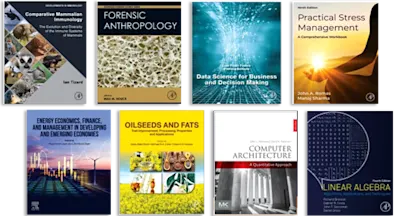
Aerodynamics for Engineering Students, Eight Edition provides concise explanations of basic concepts combined with an excellent introduction to aerodynamic theory. This updated edition has been revised with improved pedagogy and reorganized content to facilitate student learning. The book includes new examples in many chapters, expanded use of the "aerodynamics around us" boxes to help put the content into proper context for students, and more coverage and use of computational methods like MATLAB.
- Provides contemporary applications and examples that help students see the link between everyday physical examples of aerodynamics and the application of aerodynamic principles to aerodynamic design
- Contains MATLAB-based computational exercises throughout, giving students practice in using industry-standard computational tools
- Includes examples in SI and Imperial units, reflecting the fact that the aerospace industry uses both systems of units
- Includes improved pedagogy, such as more worked examples throughout, a reorganization of content, and further integration of MATLAB
Upper level undergraduate and graduate students in aeronautical engineering / Navstem estimated the US course size at 13,300 in 2020, an increase of 15% over the prior year.
1. Basic Concepts and Definitions
PART II: FUNDAMENTALS OF FLUID MECHANICS
2. Equations of Motion
3. Viscous Boundary Layers
4. Compressible Flow
PART III: AERODYNAMICS OF WINGS AND BODIES
5. Potential Flow
6. Two-Dimensional Wing Theory
7. Wing Theory
8. Airfoils and Wings in Compressible Flow
PART IV: APPLICATIONS OF AERODYNAMICS
9. Computational Fluid Dynamics
10. Flow Control, Planar and Rotating Wing Designs
Appendix
A. Symbols and Notation
B. Properties of Standard Atmosphere
C. A Solution of Glauert Type Integrals Appendix
D: Conversion of Imperial Units to Syst´eme International (SI) Units
- Edition: 8
- Published: November 22, 2024
- Imprint: Butterworth-Heinemann
- Language: English
SC
Steven H. Collicott
DV
Daniel T. Valentine
PC