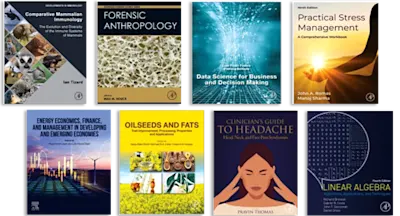
3D Printing in Analytical Chemistry
Sample Preparation, Separation, and Sensing
- 1st Edition - January 25, 2025
- Imprint: Elsevier
- Editors: José Manuel Herrero Martínez, Manuel Miró, Enrique Javier Carrasco-Correa, María Vergara-Barberán
- Language: English
- Paperback ISBN:9 7 8 - 0 - 4 4 3 - 1 5 6 7 5 - 5
- eBook ISBN:9 7 8 - 0 - 4 4 3 - 1 5 6 7 6 - 2
3D Printing in Analytical Chemistry: Sample Preparation, Separation, and Sensing covers all the applications of 3D printed systems in relevant analytical areas such as sample pr… Read more
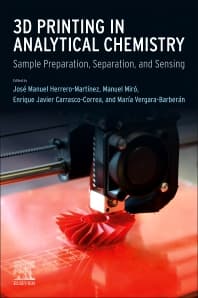
Purchase options
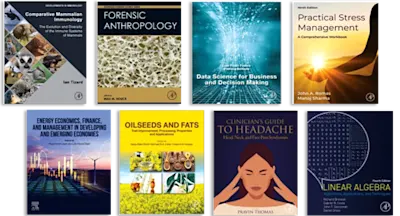
Institutional subscription on ScienceDirect
Request a sales quote3D Printing in Analytical Chemistry: Sample Preparation, Separation, and Sensing covers all the applications of 3D printed systems in relevant analytical areas such as sample preparation (use of sorbents, membranes, and devices), separation devices in analytical techniques, and as components in sensors and detection systems, among others. The book also includes key aspects on the preparation and design of novel 3D printed devices for analytical applications, including tips and tricks written by experts. Special features based on 3D printed structures for different applications are also highlighted, along with the most relevant works.
- Presents the most important features regarding 3D printing in the Analytical Chemistry field, helping researchers improve their applications
- Addresses adequate 3D printing technology for the desired application by giving tips and tricks, including the most relevant applications reported in the last years
- Provides analytical researchers with a reference compendium on the use of 3D printing in extraction, separation, and sensing methodologies
Analytical Scientists working on: 3D printing for sample preparation, separation and sensing applications; Development of new sorbents and membranes, including smart materials such as monoliths, (nano)materials, and selective molecules (antibodies, aptamers) and materials (molecularly imprinted polymers); Automation and microfluidics; Sensors; Analytical applications of different areas, including the analysis of complex samples. Academics but also Analytical Scientists and Laboratory Technicians primary related to the food, environmental, and bioanalysis sectors
- Title of Book
- Cover image
- Title page
- Table of Contents
- Copyright
- Contributors
- Abbreviations
- Abbreviation list related to 3D printing
- Section 1. 3D printing: Technology, design, and fabrication
- Chapter 1. Introduction to 3D printing from the analytical chemistry viewpoint
- 1 Role of 3D printing in analytical chemistry
- 2 3D printing for sample preparation
- 3 3D printing in analytical separation science
- 4 3D printing for (bio)sensor/detector developments
- 5 The future of 3D printing starts now
- Chapter 2. Overview of 3D printing technologies and materials
- 1 Introduction
- 1.1 Historical perspective and current situation
- 1.2 Immediate future. Trends in the period 2022–25
- 1.3 Advantages and disadvantages of 3D printing
- 1.4 Environmental commitment of 3D printing technologies
- 1.5 4D printing
- 1.6 Scope of application of 3D in different disciplines of chemistry
- 2 3D printing application
- 2.1 Characteristics of the models manufactured using 3D printing
- 2.2 Incidence sectors
- 2.3 Applications of 3D printing for analytical chemistry: General areas
- 2.3.1 Chromatography
- 2.3.2 Applications of 3D printing to extraction and preconcentration
- 2.3.3 Electrochemical applications
- 2.3.4 Microfluidic devices
- 2.3.5 Spectroscopy
- 2.3.6 Biopolymers and membranes
- 3 Work methodology
- 3.1 Design process for 3D printing
- 3.2 Component redesign for 3D printing
- 4 Analysis of 3D printing technologies
- 4.1 Classification
- 4.2 Decision variables
- 4.3 3D printing limits
- 4.3.1 Geometric modeling problems. Geometric performance
- 4.4 3D printing normalization
- 4.5 Digital spares and patent law
- 4.5.1 Qualification and certification for 3D printing
- 4.6 3D printing techniques in analytical chemistry
- 5 3D printing materials
- 5.1 Classification
- 5.2 Properties and technical specifications
- 6 Finishing of 3D-printed models
- 6.1 Classification of postprocessing techniques according to the purpose
- 6.2 Description of current postprocessing techniques
- 7 Conclusions and future perspectives
- Chapter 3. Tips and tricks for fabrication of 3D printing devices for analytical applications
- 1 Introduction
- 2 Tips and tricks
- 2.1 Fused deposition modeling strategies
- 2.2 Stereolithographic strategies
- 2.3 Photopolymer inkjet printing
- 3 Conclusions
- Chapter 4. 3D printing of analytical platforms: From prototyping to commercialization
- 1 Introduction
- 2 3D-printing market and economic trends
- 3 3D-printing for manufacturing – A matter of scale
- 4 Additive manufacturing and miniaturization
- 5 Micro and nano 3D printing advancing analytical platforms
- 6 Conclusion
- Section 2. 3D printing in analytical sample preparation
- Chapter 5. 3D-printed scaffolds for sample handling and preparation
- 1 Introduction
- 2 Scaffolds for the analytical laboratory
- 3 Postprint processing and printout modification
- 4 3D-printed scaffolds in sample preparation
- 4.1 Scaffolds for handling magnetic sorbents
- 4.2 Stirring devices for sampling and preconcentration
- 4.3 Magnetic levitation scaffolds
- 4.4 3D-printed scaffolds with immobilized sorbent
- 4.4.1 Immobilization of nonconventional sorbents for sample preparation
- 4.4.2 Immobilization of commercial resins
- 4.5 3D-printed housings and cartridges for solid-phase extractions
- 5 Scaffolds as holders in membrane-based separations
- 6 Scaffolds for instruments related to laboratory automation
- 6.1 Autosamplers, collectors, and robotic automation
- 6.2 Use in the manual preparative as well as automation approach Lab-In-Syringe
- 7 Scaffolds for paper devices
- 7.1 3D printing for paper device fabrication
- 7.2 3D-printed supports for paper devices
- 7.3 Lighting boxes
- 8 3D-printed laboratory equipment
- 8.1 Standard laboratory equipment and instruments
- 8.2 Reactionware
- 9 3D-printed scaffolds for sampling
- 10 3D-printed elements related to teaching analytical chemistry
- 11 Summarizing discussion and outlook
- Chapter 6. 3D-printed materials in solid-phase extraction and microextraction
- 1 3D printing of sorbents and extraction devices
- 2 3D-printed thermoplastic sorbents
- 2.1 FDM 3D-printed thermoplastic sorbents
- 2.2 FDM 3D-printed functionalized thermoplastic sorbents
- 2.3 SLS 3D-printed functionalized thermoplastic sorbents
- 3 3D-printed photopolymer sorbents
- 3.1 3D-printed photopolymer sorbents
- 3.2 3D-printed functionalized photopolymer sorbents
- 4 DIW 3D-printed sorbents
- 5 3D-printed stimuli-responsive sorbents
- 6 Conclusions and future perspectives
- Chapter 7. 3D printing and smart materials for sample preparation
- 1 Sample treatment, 3D printing, and smart materials
- 2 Incorporation of smart materials to 3D-printed supports
- 2.1 Postprinting functionalization strategies
- 2.2 Preprinting incorporation strategies
- 3 Applications of smart materials in 3D-printed supports for sample preparation
- 4 Conclusions and future perspectives
- Chapter 8. 3D printed devices in membrane separation
- 1 Membranes in separation science
- 1.1 A brief introduction to the varying objectives of separation science
- 2 Membrane history, science, and technology
- 2.1 History of membrane development
- 2.1.1 Membrane science and technology
- 3 Membranes in microfluidic devices
- 3.1 Initial efforts in microdevices and lab-on-a-chip devices
- 3.1.1 Membranes in PDMS and other materials
- 4 Membranes and additive manufacturing
- 4.1 Different types of additive manufacturing
- 4.1.1 Membranes in 3D-printed devices
- 5 Conclusion
- Chapter 9. Milli/microfluidic platforms using 3D-printed devices for sample analysis
- 1 Introduction
- 2 Sample pretreatment
- 2.1 Heating systems
- 2.2 Photolysis reactors
- 2.3 Enzyme reactors
- 2.4 Liquid-phase microextraction based on 3D printing
- 3 3D-printed flow components
- 3.1 3D-printed flow-through mixers
- 3.2 3D-printed injection devices
- 3.2.1 3D-printed flow detection devices
- 3.2.2 Optical detection
- 3.2.3 Electrochemical detection
- 3.2.4 Dual-mode detection
- 3.2.5 Other detection systems
- 4 Conclusion
- Section 3. 3D printing in analytical separation techniques
- Chapter 10. Potential of 3D printing for the fabrication of stationary phases
- 1 Introduction
- 2 General requirements for the fabrication of 3D-printed stationary phases
- 2.1 Requirements associated with the additive manufacturing technology
- 2.1.1 Physical properties
- 2.1.2 Chemical properties
- 3 Materials for 3D-printed stationary phases
- 3.1 Ceramic
- 3.2 Natural polymers
- 3.3 Synthetic polymers
- 4 Characterization
- 4.1 Porous characteristics
- 4.2 Flow characteristics
- 4.3 Chemical characterization
- 4.4 Characterization of adsorption behavior
- 5 Application of 3D-printed chromatography media
- 6 Conclusions and future perspectives
- Chapter 11. 3D printing in electrophoretic techniques
- 1 Introduction
- 2 Resolution requirements
- 3 Applications
- 3.1 Free-flow electrophoresis
- 3.2 Gel electrophoresis and capillary gel electrophoresis
- 3.3 Capillary zone electrophoresis
- 3.4 Epitachophoresis and isotachophoresis
- 3.5 Hyphenation accessories and interfaces
- 4 Conclusions and future perspectives
- Chapter 12. 3D printing in mass spectrometry
- 1 Introduction
- 2 3D printers and materials for mass spectrometry applications
- 2.1 Choosing the right 3D printer and material
- 2.2 Fused deposition modeling
- 2.2.1 Thermoplastic polymers
- 2.2.2 Sacrificial filament
- 2.2.3 Functionalized materials
- 2.3 Stereolithography
- 2.3.1 Photocurable polymer resins
- 2.4 InkJet and photopolymer jetting
- 2.5 Selective laser sintering
- 2.5.1 Metal alloys and ceramics
- 3 Applications of 3D printing for mass spectrometry
- 3.1 Sample preparation and separation
- 3.1.1 3D-printed sample preparation systems for mass spectrometric analysis
- 3.1.2 Online coupling of 3D-printed (bio)chemical systems to mass spectrometers
- 3.2 Ion introduction
- 3.2.1 Ion sources
- 3.2.2 Ion guides
- 3.3 3D-printed platforms
- 3.3.1 MS interfaces
- 3.3.2 Housing and integration
- 3.3.3 3D printers as platform
- 4 Conclusions and future developments
- 4.1 Role of 3D printing in R&D
- 4.2 Role of 3D printing in open science practices
- Section 4. 3D printing devices for (bio)sensing applications
- Chapter 13. 3D printing in optical (bio)sensing
- 1 Introduction
- 2 Fused deposition modeling
- 2.1 Introduction
- 2.2 Materials
- 2.3 Printing considerations
- 2.4 Advantages and disadvantages
- 3 Key examples
- 4 Stereolithography
- 4.1 Introduction
- 4.2 Materials
- 4.3 Printing considerations
- 4.4 Advantages and disadvantages
- 4.5 Key examples
- 5 Material jetting
- 5.1 Introduction
- 5.2 Materials
- 5.3 Printing considerations
- 5.4 Advantages and disadvantages
- 5.5 Key examples
- 6 Direct ink writing
- 6.1 Introduction
- 6.2 Materials
- 6.3 Printing considerations
- 6.4 Advantages and disadvantages
- 6.5 Key examples
- 7 Conclusions
- Chapter 14. 3D printing devices for infrared, Raman, and other detectors in (bio)sensing applications
- 1 Vibrational spectroscopy in (bio)sensing
- 2 3D-printing and molecular spectroscopy
- 2.1 Sample handling and treatment
- 2.2 Measurement cells and other modules or components
- 3 Applications
- 4 Conclusions and future trends
- Chapter 15. 3D-printed electrochemical sensors
- 1 Background on 3D printing technology applied for electrochemistry
- 2 Electroanalysis using 3D-printed electrodes
- 2.1 Investigation of electrode processes towards electrochemical sensing
- 2.2 Posttreatment of 3D-printed electrodes
- 2.3 Influence of 3D printing parameters on the electrode processes
- 3 Applications
- 3.1 Applications in electrochemical forensics analysis
- 3.2 Applications in electrochemical food analysis
- 3.3 Applications in electrochemical (bio)fuels analysis
- 3.4 Applications in electrochemical natural water contaminants analysis
- 4 Conclusions and perspectives
- Chapter 16. Micro/millifluidic platforms for electrochemical detection
- 1 Introduction
- 2 3D printing modes
- 3 Microfluidics using electrochemical detection
- 4 Materials for fabrication
- 5 Electrode fabrication
- 6 Applications of electrochemical detection
- 6.1 Potentiometry
- 6.2 Amperometry
- 6.3 Voltammetry
- 6.4 Conductimetry
- 6.5 Electrochemiluminescence
- 6.6 Electrochemical impedance spectroscopy
- 7 Conclusions
- Chapter 17. Micro/millifluidic platforms for bioanalytical applications
- 1 Introduction
- 2 3D printing microfluidic/millifluidic systems
- 2.1 Most 3D printing techniques used for fabrication of microfluidic/millifluidic devices
- 2.1.1 Extrusion-based 3D printing
- 2.1.2 Polymerization-based 3D printing
- 2.1.3 Comparison of the techniques
- 3 Bioanalytical applications
- 3.1 Biological sample pretreatment and preparation
- 3.2 Enzymatic sensors
- 3.3 Immuno- and affinity sensors
- 3.4 Bacterial detection
- 3.5 Wearable sensors (metabolites detection)
- 3.5.1 Wearable sensors for the detection of glucose and lactate
- 3.5.2 Wearable sensors for the detection of electrolytes
- 4 Conclusions and perspectives/challenges
- Section 5. Perspectives on 3D printing
- Chapter 18. 3D printing in analytical chemistry—Quo Vadis?
- 1 Introduction
- 2 Definitions and selection criteria for the processed data
- 3 Advanced analysis of publications
- 3.1 Group 1: Devices that are not in contact with the sample and simple parts
- 3.2 Group 2: Devices contacting sample without complex geometry and specific physical and chemical properties
- 3.3 Group 3: Devices with complex geometry (but without specific physical and chemical properties)
- 3.4 Group 4: Devices with specific physical properties
- 3.5 Group 5: Devices with specific chemical properties
- 3.5.1 Electrodes
- 3.5.2 Solid-phase extraction
- 3.5.3 Surface modification and formation of monoliths in 3D-printed channels
- 3.5.4 Multimaterial printed items
- 4 Conclusions and future perspectives
- Index
- Edition: 1
- Published: January 25, 2025
- Imprint: Elsevier
- No. of pages: 498
- Language: English
- Paperback ISBN: 9780443156755
- eBook ISBN: 9780443156762
JH
José Manuel Herrero Martínez
Dr. José Manuel Herrero Martínez is Full Professor at the Dept. of Analytical Chemistry, University of Valencia, Spain (2018-). He obtained his PhD in Chemistry from Univ. Valencia (2000) with a Doctoral Extraordinary Award. He has worked as assistant professor (2001–2005) at Department of Analytical Chemistry (Univ. Barcelona, Spain) and as a post-doctoral researcher (2003–2004) at Department of Chemical Engineering (Univ. of Amsterdam, The Netherlands). He has carried out postdoctoral research at several institutions, such as the National University of La Plata, Argentina and Paris-East Créteil University, France. He has published around 200 research articles in international indexed journals (most of them located in Q1), and several book chapters (h-index 36, over 4900 citations, Scopus). He has presented more than 350 communications at numerous international and national scientific meetings under different formats. He has led/participated in regional, national and international R&D projects (> 20). He has supervised 11 doctoral theses, and an additional four PhD theses are currently in progress. He has collaborated with research groups of several national and foreign universities. His research interests comprise the development of novel stationary phases for separation techniques and smart materials and 3D printing for sample preparation purposes.
MM
Manuel Miró
Dr. Manuel Miró received his M.Sc. (1998) and Ph.D. (2002) in Chemistry at the University of the Balearic Islands, Spain. He has conducted post-doctoral research in several universities including the Technical University of Berlin, Technical University of Denmark and University of Natural Resources and Applied Life Sciences in Austria. He is currently Full Professor in Analytical Chemistry (from September 2017) at the University of the Balearic Islands; Visiting Professor at Charles University (Czech Republic); and member of the IUPAC Chemistry and Environment Division (Subcommittee on Chemical and Biophysical Processes in the Environment). He is the Reviews Editor of the journal Analytica Chimica Acta (Elsevier, IF:5.7, second highest IF across multi-scope analytical chemistry journals) and Associate Editor of the Encyclopaedia of Analytical Science, 3rd Edition, Elsevier, since 2007 and 2016, respectively. His publication record shows over 230 refereed articles including 16 book chapters, with an h-index of 44 and over 6500 citations and is corresponding author of over 116 articles. He has published 26 articles in Analytical Chemistry from ACS. He has delivered over 74 oral presentations (65 as opening, keynote or invited lecturer) in international conferences on analytical chemistry, sample preparation, nanotechnology, environmental chemistry, and automation based on flow methodology. He has presented over 200 poster communications in international conferences and symposiums. He supervised 10 PhD students (+ 3 ongoing) in national and international universities (Technical University of Denmark, Mahidol University in Thailand, University of the Balearic Islands and Universidade Federal de Bahia in Brasil). He has been actively engaged in 38 national and international research projects (e.g., University of Melbourne and Charles University in Czech Republic) 18 of which as the Principal Investigator. His research interests are focused on the development of on-line sample processing strategies for isolation and/or preconcentration of trace levels of environmental pollutants exploiting the various generations of flow analysis, including Lab-on-a-Valve platforms and 3-D printed fluidic structures, in hyphenation with modern analytical instrumentation.
EC
Enrique Javier Carrasco-Correa
Dr. Enrique Carrasco-Correa is an Associate Professor of Analytical Chemistry at the University of Valencia, Spain. He earned his Ph.D. in Chemistry from the University of Valencia in 2015. His research interests span a broad spectrum of analytical techniques and their applications, including capillary (electro)separation techniques, development of novel stationary phases for separation techniques, sample preparation methodologies, biomimetic systems, and, more recently, advancing the use of 3D printing in analytical chemistry. Dr. Carrasco-Correa has published approximately 45 research articles in international indexed journals, with the majority appearing in first-quartile publications. He has also contributed three book chapters. His work has garnered 701 citations, reflecting an h-index of 16 (Scopus). He has presented over 100 communications at international and national scientific meetings across various formats and has participated in the organization of two research symposiums (one national and one international) and four outreach symposiums. He has participated in 19 regional, national, and international R&D projects, including technology transfer initiatives, where he has served as principal investigator for two regional projects. Additionally, Dr. Carrasco-Correa has been involved in 65 collaboration agreements with companies from diverse fields such as detergency and agri-food, significantly advancing technology transfer. Notably, he co-developed a patented application of nanoparticles in agriculture in collaboration with Agrostock S.A. He has worked closely with research groups at various national and international universities and has actively participated in research networks focused on sample preparation in Analytical Chemistry.
MV
María Vergara-Barberán
Dr. María Vergara-Barberán is Assistant Professor in Analytical Chemistry at the University of Valencia, Spain (2023-). She received her Ph.D. (2017) in Chemistry at the University of Valencia. Her research interests comprise a wide spectrum of analytical techniques and applications including chromatographic and capillary (electro)separation techniques, mass spectrometry, preparation of novel materials for microextraction techniques including proteins, aptamers and 3D printed systems. She has published around 41 research articles in international indexed journals (most of them located in the first quartile), and 8 book chapters (635 citations, h-index 15, Scopus). She has presented more than 95 communications at numerous international and national scientific meetings under different formats. He has participated in regional, national and international R&D projects (9). Besides, she has collaborated with research groups of several national and foreign universities.